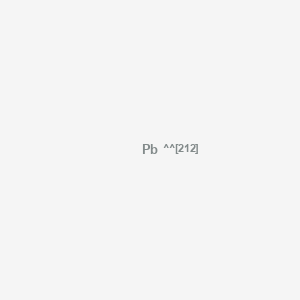
Lead, isotope of mass 212
- Click on QUICK INQUIRY to receive a quote from our team of experts.
- With the quality product at a COMPETITIVE price, you can focus more on your research.
Overview
Description
Lead-212 (²¹²Pb) is a radioactive isotope of lead with a half-life of 10.6 hours, decaying via beta-minus emission (β⁻) to bismuth-212 (²¹²Bi) and subsequently emitting alpha particles (α) as part of the thorium decay series . It is produced from the decay of thorium-228 (²²⁸Th), which is derived from neutron-irradiated radium-226 (²²⁶Ra) . ²¹²Pb has gained prominence in nuclear medicine due to its alpha-emitting daughter isotopes (²¹²Bi/²¹²Po), which enable Targeted Alpha Therapy (TAT) for cancer treatment. Its short half-life ensures minimal residual radiation exposure post-treatment, making it ideal for clinical applications .
Q & A
Basic Questions
Q. What are the primary nuclear properties of ²¹²Pb that make it relevant for medical and experimental research?
- ²¹²Pb decays via β⁻ emission (half-life: 10.64 hours) to ²¹²Bi, which subsequently emits α-particles. This dual decay pathway enables its use in Targeted Alpha Therapy (TAT), where localized α-radiation damages cancer cells while minimizing systemic toxicity. The short half-life necessitates efficient production and purification protocols .
- Key Data :
Property | Value |
---|---|
Decay mode | β⁻ to ²¹²Bi |
Half-life | 10.64 hours |
Radiation type | β⁻, α (via ²¹²Bi) |
Q. How is ²¹²Pb produced, and what are the challenges in scaling its availability for preclinical studies?
- ²¹²Pb is derived from the decay chain of ²²⁸Th (thorium-228), which is neutron-irradiated ²²⁶Ra. Extraction methods include direct isolation from ²²⁸Th or via the intermediate isotope ²²⁴Ra (radium-224). Challenges include handling high-activity α-emitters safely and optimizing separation techniques to minimize isotopic impurities .
- Methodology :
- Use shielded hot cells and radiochemical purification (e.g., ion-exchange chromatography) to isolate ²¹²Pb .
Q. What safety protocols are critical when handling ²¹²Pb in laboratory settings?
- Alpha-emitting isotopes require radiological containment (e.g., gloveboxes, negative-pressure hot cells) to prevent inhalation or contamination. Dose monitoring, waste management, and adherence to Good Manufacturing Practices (GMP) are essential for clinical-grade production .
Advanced Research Questions
Q. How can researchers reconcile discrepancies in reported half-life values or decay branching ratios for ²¹²Pb?
- Discrepancies may arise from measurement techniques (e.g., gamma spectroscopy vs. mass spectrometry) or impurities in samples. Cross-validation using standardized reference materials and decay-chain modeling (e.g., Bateman equations) can resolve inconsistencies. Peer-reviewed nuclear databases (e.g., IUPAC) provide consensus values .
- Example Workflow :
Calibrate detectors with certified ²¹²Pb sources.
Perform decay energy analysis using high-purity germanium (HPGe) detectors .
Q. What experimental design considerations are vital for optimizing ²¹²Pb-based radiopharmaceuticals in preclinical models?
- Key factors include:
- Targeting molecule stability : Ensure the chelator (e.g., DOTA, TCMC) retains ²¹²Pb under physiological conditions.
- Biodistribution studies : Use SPECT/CT imaging to track ²¹²Pb-²¹²Bi in vivo, accounting for daughter nuclide redistribution .
- Dosimetry : Calculate absorbed doses using Monte Carlo simulations to balance efficacy and toxicity .
Q. How can isotopic impurities (e.g., ²¹⁰Pb) impact experimental outcomes, and what purification strategies mitigate this?
- Long-lived impurities like ²¹⁰Pb (half-life: 22.3 years) introduce background radiation and confound data. Mitigation involves:
- Chromatographic purification : Optimize elution parameters to separate Pb isotopes based on ionic radius.
- Quality control : Use alpha-particle spectroscopy to verify purity (>99%) before experimentation .
Q. What statistical methods are recommended for analyzing conflicting datasets in ²¹²Pb decay studies?
- Apply Bayesian inference or error-weighted averaging to integrate data from multiple labs. Report uncertainties using the Guide to the Expression of Uncertainty in Measurement (GUM) framework. For example, discrepancies in half-life measurements (e.g., 10.6 vs. 10.7 hours) should be evaluated for systematic vs. random errors .
Q. Data Analysis and Reporting
Q. How should researchers handle the short half-life of ²¹²Pb in time-sensitive experiments?
- Use real-time activity correction (decay factor: e−λt) during data acquisition. Schedule experiments within 2–3 half-lives (20–30 hours) to maintain detectable activity. Pre-calibrate equipment to minimize setup delays .
Q. What are best practices for publishing ²¹²Pb-related data to ensure reproducibility?
- Include:
Comparison with Similar Compounds
Comparison with Similar Isotopes and Compounds
Lead-212 vs. Other Lead Isotopes
Lead isotopes vary in stability, decay modes, and applications. A comparison with key isotopes is summarized below:
Key Differences :
- Stability : ²¹²Pb is synthetic and highly radioactive, unlike stable isotopes (²⁰⁴Pb–²⁰⁸Pb) used in environmental or geological studies .
- Medical Utility : ²¹²Pb’s alpha emissions contrast with stable isotopes’ roles in analytical chemistry (e.g., blood lead quantification via ICP-MS ).
- Decay Chain: ²¹²Pb’s decay produces therapeutic alpha particles, whereas ²¹⁰Pb (longer-lived) is used for sediment chronology .
Lead-212 vs. Other Alpha-Emitting Isotopes
²¹²Pb is compared to isotopes used in TAT and diagnostic radiopharmaceuticals:
Key Insights :
- Production Complexity : ²¹²Pb requires advanced nuclear facilities (e.g., shielded hot cells) for safe extraction from ²²⁸Th/²²⁴Ra, whereas ²²⁵Ac production involves cyclotron-based methods .
- Therapeutic Window : ²¹²Pb’s 10.6-hour half-life balances tumor targeting and clearance, outperforming shorter-lived isotopes (e.g., ²¹³Bi) and reducing logistical challenges compared to ²²⁴Ra .
Analytical Methods for Isotopic Characterization
Accurate measurement of ²¹²Pb and related isotopes relies on:
- ICP-MS : Used for quantifying lead isotopes in biological samples (e.g., blood) with precision (RSD <1%) .
- Isotope Pattern Deconvolution : Separates isotopic signatures in complex matrices (e.g., environmental water samples) .
- Alpha Spectrometry : Critical for validating ²¹²Pb purity in radiopharmaceuticals .
Research Findings and Challenges
- Medical Efficacy : Preclinical studies highlight ²¹²Pb’s efficacy in treating metastatic cancers, with clinical trials underway to optimize dosing .
- Supply Chain Limitations: Limited availability of ²²⁸Th/²²⁴Ra sources and high production costs hinder large-scale ²¹²Pb adoption .
- Safety Protocols : Handling ²¹²Pb requires GMP-compliant facilities to mitigate alpha radiation risks .
Preparation Methods
Generator-Based Production Systems
Generator systems remain the most widely adopted method for ²¹²Pb production, leveraging the decay chains of parent isotopes such as thorium-228 (²²⁸Th) and radium-224 (²²⁴Ra). These systems balance practicality with the need for periodic elution of ²¹²Pb, ensuring a continuous supply for clinical applications .
The ²²⁸Th/²²⁴Ra/²¹²Pb Decay Chain
The ²²⁸Th decay series provides a robust framework for ²¹²Pb generation. ²²⁸Th (half-life: 1.91 years) decays sequentially to ²²⁴Ra (3.66 days), ²²⁰Rn (55.6 seconds), and ²¹²Pb via alpha emissions . A generator utilizing this chain typically employs a two-step process:
-
Parent Isotope Immobilization : ²²⁸Th is adsorbed onto a chromatographic resin, such as strong acid cation exchange (e.g., AG MP-50), to minimize breakthrough during elution .
-
²¹²Pb Elution : The gaseous ²²⁰Rn produced from ²²⁴Ra decay is transported via an inert gas stream to a collection chamber, where it decays to ²¹²Pb. The ²¹²Pb is then purified using anion exchange resins (e.g., Dowex 1X8) with 2 M HCl, achieving yields exceeding 90% .
Key Advancements :
-
Telix Pharmaceuticals recently validated a sealed ²²⁸Th generator system that automates ²¹²Pb elution, producing up to 60 clinical doses per cycle with <0.04% parent isotope breakthrough .
-
Multicolumn selectivity inversion (MCSIG) systems have enhanced purity by sequentially removing ²²⁴Ra and ²²⁸Th contaminants through LN-2 and UTEVA resins, achieving decontamination factors >10⁸ .
The ²²⁴Ra/²¹²Pb Generator
This generator design bypasses ²²⁸Th by directly utilizing ²²⁴Ra as the parent isotope. ²²⁴Ra is immobilized on a cation exchange resin, and ²¹²Pb is eluted using 0.5–2 M HCl. However, challenges include:
-
Radiolysis : Prolonged exposure to alpha radiation degrades the resin, increasing ²²⁴Ra leakage over time .
-
Yield Limitations : Traditional systems yield ~600 MBq (16 mCi) per elution, necessitating frequent generator replacement .
Recent Innovations :
-
Oak Ridge National Laboratory developed a high-capacity ²²⁴Ra/²¹²Pb generator using MP50 resin, achieving 95% ²¹²Pb recovery with <1 ppm ²²⁴Ra contamination .
-
Automated elution systems now integrate real-time activity monitoring, optimizing elution intervals for clinical demand .
Direct Production via Photonuclear Reactions
Photoconversion of ²²⁶Ra in linear accelerators offers an alternative to generator-dependent production. The reaction ²²⁶Ra(γ,2n)²²⁴Ra → ²¹²Pb generates ²¹²Pb as a byproduct of ²²⁵Ac synthesis .
Process Parameters
-
Energy Threshold : A 35–45 MeV electron beam irradiates a ²²⁶Ra target, inducing photonuclear reactions .
-
Separation Challenges : Co-produced ²²⁵Ac requires intricate purification via solvent extraction (e.g., DGA resin), reducing ²¹²Pb yields .
Scalability :
-
IBA Industrial Solutions’ Rhodotron accelerators can produce multi-Ci quantities of ²²⁵Ac, indirectly yielding ²¹²Pb at ~6:1 ratio .
Industrial-Scale Production and Purification
Telix’s Automated Generator System
Telix’s 2025 breakthrough integrates a sealed ²²⁸Th source with a fully automated elution platform, addressing historical limitations:
Parameter | Telix Generator | Traditional Generator |
---|---|---|
Activity per Elution | 12 GBq | 600 MBq |
Parent Breakthrough | <0.001% | <1% |
Dose Capacity | 60 | 10 |
This system employs a thorium stearate matrix to enhance ²²⁰Rn emanation, coupled with continuous gas flow for ²¹²Pb deposition .
Chromatographic Purification
Post-elution purification is critical for clinical-grade ²¹²Pb. A three-step protocol is widely adopted:
-
Cation Exchange : Removes residual ²²⁴Ra using Dowex 50WX8 .
-
Anion Exchange : Eliminates thorium fragments via Dowex 1X2 .
-
Final Filtration : 0.22 µm sterilization ensures injectable purity .
Comparative Analysis of Production Methods
Table 1: Key Metrics for ²¹²Pb Preparation Methods
Method | Parent Isotope | Yield (%) | Radionuclide Purity | Scalability |
---|---|---|---|---|
²²⁸Th Generator | ²²⁸Th | 90 | 99.9% | Industrial |
²²⁴Ra Generator | ²²⁴Ra | 75 | 99.5% | Clinical |
Photoconversion | ²²⁶Ra | 30 | 99.0% | Pilot |
Insights :
Properties
CAS No. |
15092-94-1 |
---|---|
Molecular Formula |
P |
Molecular Weight |
211.9919 g/mol |
IUPAC Name |
lead-212 |
InChI |
InChI=1S/Pb/i1+5 |
InChI Key |
WABPQHHGFIMREM-BKFZFHPZSA-N |
SMILES |
[Pb] |
Isomeric SMILES |
[212Pb] |
Canonical SMILES |
[Pb] |
Synonyms |
212Pb radioisotope Lead-212 Pb-212 radioisotope |
Origin of Product |
United States |
Disclaimer and Information on In-Vitro Research Products
Please be aware that all articles and product information presented on BenchChem are intended solely for informational purposes. The products available for purchase on BenchChem are specifically designed for in-vitro studies, which are conducted outside of living organisms. In-vitro studies, derived from the Latin term "in glass," involve experiments performed in controlled laboratory settings using cells or tissues. It is important to note that these products are not categorized as medicines or drugs, and they have not received approval from the FDA for the prevention, treatment, or cure of any medical condition, ailment, or disease. We must emphasize that any form of bodily introduction of these products into humans or animals is strictly prohibited by law. It is essential to adhere to these guidelines to ensure compliance with legal and ethical standards in research and experimentation.