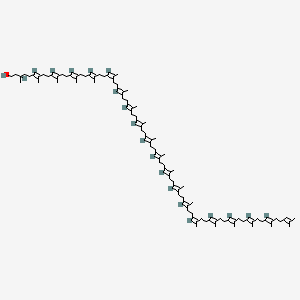
Dolichol-20
Overview
Description
Dolichol-20 is a long-chain polyisoprenoid alcohol consisting of 20 isoprene units. It is a member of the dolichol family, which plays a crucial role in the post-translational modification of proteins, particularly in the process of N-glycosylation. This compound is involved in the synthesis of glycoproteins by acting as a lipid carrier for sugar molecules in the endoplasmic reticulum of eukaryotic cells .
Preparation Methods
Synthetic Routes and Reaction Conditions: Dolichol-20 is synthesized through the mevalonate pathway, which involves several enzymatic steps. The process begins with the condensation of acetyl-CoA to form 3-hydroxy-3-methylglutaryl-CoA (HMG-CoA), which is then reduced to mevalonate by HMG-CoA reductase. Mevalonate undergoes phosphorylation and decarboxylation to produce isopentenyl pyrophosphate (IPP). IPP is then converted to farnesyl pyrophosphate (FPP), which serves as the precursor for dolichol synthesis. The enzyme cis-prenyltransferase catalyzes the polymerization of FPP with additional IPP units to form polyprenyl pyrophosphate, which is subsequently reduced to this compound .
Industrial Production Methods: Industrial production of this compound involves the fermentation of yeast or bacterial cultures engineered to overproduce the enzymes required for the mevalonate pathway. The cultures are grown in bioreactors under controlled conditions to maximize the yield of this compound. The compound is then extracted and purified using chromatographic techniques .
Chemical Reactions Analysis
Types of Reactions: Dolichol-20 undergoes various chemical reactions, including:
Oxidation: this compound can be oxidized to form dolichol phosphate, which is essential for glycoprotein synthesis.
Reduction: The reduction of this compound involves the conversion of polyprenol to dolichol by the enzyme polyprenol reductase.
Substitution: this compound can participate in substitution reactions where the hydroxyl group is replaced by other functional groups.
Common Reagents and Conditions:
Oxidation: Common oxidizing agents include molecular oxygen and hydrogen peroxide.
Reduction: Reducing agents such as sodium borohydride or lithium aluminum hydride can be used.
Substitution: Reagents like acyl chlorides or alkyl halides are commonly used in substitution reactions.
Major Products:
Dolichol Phosphate: Formed through the oxidation of this compound.
Glycosylated Dolichol: Resulting from the attachment of sugar molecules during glycoprotein synthesis.
Scientific Research Applications
Dolichol-20 has several scientific research applications, including:
Chemistry: Used as a model compound to study the properties and reactions of polyisoprenoids.
Biology: Plays a critical role in the study of protein glycosylation and cellular processes involving lipid carriers.
Medicine: Investigated for its potential role in aging and neurodegenerative diseases, as changes in dolichol levels are associated with conditions like Alzheimer’s disease.
Industry: Utilized in the production of glycoproteins and other biotechnological applications .
Mechanism of Action
Dolichol-20 exerts its effects primarily through its role in protein glycosylation. It acts as a lipid carrier for the assembly and transfer of oligosaccharides to nascent proteins in the endoplasmic reticulum. The process involves the formation of dolichol-linked oligosaccharides, which are then transferred to specific asparagine residues on the target proteins by the enzyme oligosaccharyltransferase. This modification is crucial for proper protein folding, stability, and function .
Comparison with Similar Compounds
Dolichol-20 is compared with other similar compounds such as:
Dolichol-15: Contains 15 isoprene units and has similar functions but differs in chain length and specific biological roles.
Dolichol-25: Contains 25 isoprene units and is involved in similar glycosylation processes but may have different cellular distributions and functions.
Polyprenol: The precursor to dolichol, which is reduced to form this compound. .
This compound is unique due to its specific chain length, which influences its role in glycosylation and its distribution within cellular membranes. Its distinct properties make it a valuable compound for studying lipid-mediated processes in cells .
Properties
IUPAC Name |
(6E,10E,14E,18E,22Z,26E,30E,34E,38E,42E,46E,50E,54E,58Z,62E,66E,70E,74E)-3,7,11,15,19,23,27,31,35,39,43,47,51,55,59,63,67,71,75,79-icosamethyloctaconta-6,10,14,18,22,26,30,34,38,42,46,50,54,58,62,66,70,74,78-nonadecaen-1-ol | |
---|---|---|
Source | PubChem | |
URL | https://pubchem.ncbi.nlm.nih.gov | |
Description | Data deposited in or computed by PubChem | |
InChI |
InChI=1S/C100H164O/c1-81(2)41-22-42-82(3)43-23-44-83(4)45-24-46-84(5)47-25-48-85(6)49-26-50-86(7)51-27-52-87(8)53-28-54-88(9)55-29-56-89(10)57-30-58-90(11)59-31-60-91(12)61-32-62-92(13)63-33-64-93(14)65-34-66-94(15)67-35-68-95(16)69-36-70-96(17)71-37-72-97(18)73-38-74-98(19)75-39-76-99(20)77-40-78-100(21)79-80-101/h41,43,45,47,49,51,53,55,57,59,61,63,65,67,69,71,73,75,77,100-101H,22-40,42,44,46,48,50,52,54,56,58,60,62,64,66,68,70,72,74,76,78-80H2,1-21H3/b82-43+,83-45+,84-47+,85-49+,86-51-,87-53+,88-55+,89-57+,90-59+,91-61+,92-63+,93-65+,94-67+,95-69-,96-71+,97-73+,98-75+,99-77+ | |
Source | PubChem | |
URL | https://pubchem.ncbi.nlm.nih.gov | |
Description | Data deposited in or computed by PubChem | |
InChI Key |
KEVPZUBEAUSPNJ-OYHKHEHLSA-N | |
Source | PubChem | |
URL | https://pubchem.ncbi.nlm.nih.gov | |
Description | Data deposited in or computed by PubChem | |
Canonical SMILES |
CC(CCC=C(C)CCC=C(C)CCC=C(C)CCC=C(C)CCC=C(C)CCC=C(C)CCC=C(C)CCC=C(C)CCC=C(C)CCC=C(C)CCC=C(C)CCC=C(C)CCC=C(C)CCC=C(C)CCC=C(C)CCC=C(C)CCC=C(C)CCC=C(C)CCC=C(C)C)CCO | |
Source | PubChem | |
URL | https://pubchem.ncbi.nlm.nih.gov | |
Description | Data deposited in or computed by PubChem | |
Isomeric SMILES |
CC(CC/C=C(\C)/CC/C=C(\C)/CC/C=C(\C)/CC/C=C(\C)/CC/C=C(/C)\CC/C=C(\C)/CC/C=C(\C)/CC/C=C(\C)/CC/C=C(\C)/CC/C=C(\C)/CC/C=C(\C)/CC/C=C(\C)/CC/C=C(\C)/CC/C=C(/C)\CC/C=C(\C)/CC/C=C(\C)/CC/C=C(\C)/CC/C=C(\C)/CCC=C(C)C)CCO | |
Source | PubChem | |
URL | https://pubchem.ncbi.nlm.nih.gov | |
Description | Data deposited in or computed by PubChem | |
Molecular Formula |
C100H164O | |
Source | PubChem | |
URL | https://pubchem.ncbi.nlm.nih.gov | |
Description | Data deposited in or computed by PubChem | |
Molecular Weight |
1382.4 g/mol | |
Source | PubChem | |
URL | https://pubchem.ncbi.nlm.nih.gov | |
Description | Data deposited in or computed by PubChem | |
Physical Description |
Solid | |
Record name | Dolichol-20 | |
Source | Human Metabolome Database (HMDB) | |
URL | http://www.hmdb.ca/metabolites/HMDB0005176 | |
Description | The Human Metabolome Database (HMDB) is a freely available electronic database containing detailed information about small molecule metabolites found in the human body. | |
Explanation | HMDB is offered to the public as a freely available resource. Use and re-distribution of the data, in whole or in part, for commercial purposes requires explicit permission of the authors and explicit acknowledgment of the source material (HMDB) and the original publication (see the HMDB citing page). We ask that users who download significant portions of the database cite the HMDB paper in any resulting publications. | |
CAS No. |
2067-66-5 | |
Record name | Dolichol | |
Source | ChemIDplus | |
URL | https://pubchem.ncbi.nlm.nih.gov/substance/?source=chemidplus&sourceid=0002067665 | |
Description | ChemIDplus is a free, web search system that provides access to the structure and nomenclature authority files used for the identification of chemical substances cited in National Library of Medicine (NLM) databases, including the TOXNET system. | |
Record name | Dolichol-20 | |
Source | Human Metabolome Database (HMDB) | |
URL | http://www.hmdb.ca/metabolites/HMDB0005176 | |
Description | The Human Metabolome Database (HMDB) is a freely available electronic database containing detailed information about small molecule metabolites found in the human body. | |
Explanation | HMDB is offered to the public as a freely available resource. Use and re-distribution of the data, in whole or in part, for commercial purposes requires explicit permission of the authors and explicit acknowledgment of the source material (HMDB) and the original publication (see the HMDB citing page). We ask that users who download significant portions of the database cite the HMDB paper in any resulting publications. | |
Retrosynthesis Analysis
AI-Powered Synthesis Planning: Our tool employs the Template_relevance Pistachio, Template_relevance Bkms_metabolic, Template_relevance Pistachio_ringbreaker, Template_relevance Reaxys, Template_relevance Reaxys_biocatalysis model, leveraging a vast database of chemical reactions to predict feasible synthetic routes.
One-Step Synthesis Focus: Specifically designed for one-step synthesis, it provides concise and direct routes for your target compounds, streamlining the synthesis process.
Accurate Predictions: Utilizing the extensive PISTACHIO, BKMS_METABOLIC, PISTACHIO_RINGBREAKER, REAXYS, REAXYS_BIOCATALYSIS database, our tool offers high-accuracy predictions, reflecting the latest in chemical research and data.
Strategy Settings
Precursor scoring | Relevance Heuristic |
---|---|
Min. plausibility | 0.01 |
Model | Template_relevance |
Template Set | Pistachio/Bkms_metabolic/Pistachio_ringbreaker/Reaxys/Reaxys_biocatalysis |
Top-N result to add to graph | 6 |
Feasible Synthetic Routes
Disclaimer and Information on In-Vitro Research Products
Please be aware that all articles and product information presented on BenchChem are intended solely for informational purposes. The products available for purchase on BenchChem are specifically designed for in-vitro studies, which are conducted outside of living organisms. In-vitro studies, derived from the Latin term "in glass," involve experiments performed in controlled laboratory settings using cells or tissues. It is important to note that these products are not categorized as medicines or drugs, and they have not received approval from the FDA for the prevention, treatment, or cure of any medical condition, ailment, or disease. We must emphasize that any form of bodily introduction of these products into humans or animals is strictly prohibited by law. It is essential to adhere to these guidelines to ensure compliance with legal and ethical standards in research and experimentation.