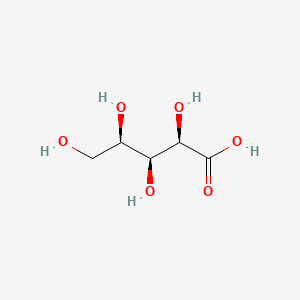
D-xylonic acid
Overview
Description
D-xylonic acid is a naturally occurring compound formed in the first step of the oxidative metabolism of D-xylose by certain archaea and bacteria.
Preparation Methods
Synthetic Routes and Reaction Conditions: D-xylonic acid can be produced through the microbial conversion of D-xylose. This process involves the action of D-xylose dehydrogenase, which converts D-xylose to this compound. Various microorganisms, including Gluconobacter oxydans and Pseudomonas putida, have been reported to produce high extracellular concentrations of this compound .
Industrial Production Methods: One of the most promising and cost-effective methods for industrial production of this compound is through microbial fermentation using inexpensive lignocellulosic feedstock. For instance, Gluconobacter oxydans has been genetically modified to overexpress the membrane-bound glucose dehydrogenase gene, resulting in significantly improved this compound production from corn stover hydrolysate .
Chemical Reactions Analysis
Types of Reactions: D-xylonic acid undergoes various chemical reactions, including oxidation, reduction, and substitution. It can act as both a solvent and a biocatalyst in three-component reactions, such as the synthesis of 3,4-dihydropyrimidin-2(1H)-ones/thiones from aldehydes, β-dicarbonyl compounds, and urea or thiourea .
Common Reagents and Conditions: Common reagents used in reactions involving this compound include aldehydes, β-dicarbonyl compounds, and urea or thiourea. The reactions are typically carried out under mild conditions, making this compound an environmentally friendly and efficient catalyst .
Major Products: Major products formed from reactions involving this compound include 3,4-dihydropyrimidin-2(1H)-ones/thiones and their derivatives, which are valuable in various industrial applications .
Scientific Research Applications
Industrial Applications
D-xylonic acid is recognized as a valuable platform chemical with several industrial applications:
- Concrete Dispersant : this compound is utilized as a dispersant in concrete formulations. It enhances the fluidity and workability of concrete mixtures, allowing for improved dispersion of solid particles and reducing water requirements, which can lead to stronger concrete structures .
- Chelating Agent : The compound acts as an effective chelator, binding metal ions and thereby improving the stability and performance of various formulations in pharmaceuticals and agriculture .
- Precursor for Polymers : this compound serves as a precursor for the synthesis of bio-based polymers, including co-polyamides and polyesters. These materials are increasingly important in developing sustainable alternatives to petroleum-based plastics .
- Food Industry Applications : In food science, this compound is explored for its potential to reduce acrylamide formation during cooking processes, thus enhancing food safety. Additionally, it is used as an additive to improve food texture and shelf-life .
Biotechnological Applications
The biotechnological potential of this compound is significant, particularly in microbial fermentation processes:
- Microbial Production : Engineered strains of Escherichia coli and other microorganisms have been developed to produce high yields of this compound from D-xylose. For instance, modifications in E. coli have led to production levels exceeding 39 g/L under optimized conditions . This bioconversion process offers a sustainable alternative to chemical synthesis methods.
- Genetic Engineering : Recent advancements include the development of pH-responsive genetic sensors that regulate this compound accumulation during microbial fermentation. This innovation addresses challenges related to culture media acidification caused by intermediate buildup .
Pharmaceutical Applications
This compound's role in pharmaceuticals is emerging due to its properties as a biocatalyst:
- Biocatalyst in Organic Synthesis : Research indicates that this compound can act as an effective biocatalyst in three-component reactions for synthesizing pharmaceutical intermediates like pyrrole derivatives. This application highlights its potential in green chemistry by facilitating reactions under mild conditions .
Table 1: Summary of Research Findings on this compound Applications
Mechanism of Action
D-xylonic acid exerts its effects through its role in the oxidative metabolism of D-xylose. The compound is formed by the action of D-xylose dehydrogenase, which converts D-xylose to this compound. This process is part of the xylose oxidative pathway, which has been engineered in microorganisms for the production of various industrially relevant compounds .
Comparison with Similar Compounds
D-gluconic acid: Like D-xylonic acid, D-gluconic acid is a sugar acid derived from glucose.
D-glucaric acid: Another sugar acid, D-glucaric acid, is derived from glucose and has applications in the production of biodegradable polymers and other industrial products.
Uniqueness of this compound: this compound is unique in its ability to be produced from non-food carbohydrate sources, making it an attractive alternative to other sugar acids like D-gluconic acid. Its versatility as a platform chemical and its broad range of applications in various fields further highlight its uniqueness .
Biological Activity
D-xylonic acid, a sugar acid derived from xylose, has garnered attention due to its potential applications in biotechnology and its biological activity. This article explores the biological activity of this compound, focusing on its production, metabolic pathways, and effects on microbial systems.
Overview of this compound
This compound is produced through the oxidation of D-xylose, primarily by certain bacteria and archaea. The metabolic pathway involves the enzyme D-xylose dehydrogenase (D-XDH), which converts D-xylose into D-xylonolactone, subsequently hydrolyzed to form this compound. This process is significant in microbial fermentation, where engineered strains are developed for enhanced production.
Production Mechanisms
The most common method for producing this compound is microbial fermentation. Various microorganisms have been genetically modified to optimize this process:
- Saccharomyces cerevisiae : Engineered with D-XDH from Hypocrea jecorina and Caulobacter crescentus, resulting in improved yields of D-xylonate.
- Kluyveromyces lactis : Similar modifications have been applied to enhance production rates.
Table 1 summarizes some key studies on the production of this compound:
Toxicity and Accumulation
The accumulation of this compound in fermentation processes can lead to media acidification, which negatively impacts microbial growth and enzymatic activity. This phenomenon is particularly problematic in engineered strains utilizing the xylose oxidative pathway (XOP). Studies indicate that the overexpression of xylose dehydrogenase contributes to this accumulation, causing toxic effects that hinder product formation .
Genetic Engineering Approaches
To mitigate the adverse effects associated with this compound accumulation, several genetic engineering strategies have been employed:
- Enzyme Engineering : Modifying enzymes involved in the xylose metabolic pathway to enhance their efficiency.
- Evolutionary Engineering : Selecting strains with better tolerance to acidic conditions.
- Coutilization of Sugars : Utilizing alternative sugar substrates alongside xylose to balance metabolic byproducts.
These strategies aim to improve the overall yield of desired products while minimizing the negative impact of this compound accumulation .
Case Study 1: Enhanced Production in S. cerevisiae
In a study by Toivari et al. (2012), researchers demonstrated that expressing the xylB gene from C. crescentus in S. cerevisiae significantly increased D-xylonate production rates compared to control strains. The engineered strain produced up to 43 g/L at a rate of approximately 0.5 g/L/h, showcasing the effectiveness of genetic modifications in optimizing metabolic pathways .
Case Study 2: Biotransformation in Pseudomonas putida
Dvořák et al. (2020) explored the biotransformation of D-xylose to this compound using Pseudomonas putida. The study found that under optimal conditions, the strain produced 2.75 g/L of xylonate from xylose while maintaining cellular growth, indicating a potential for industrial applications .
Q & A
Basic Research Questions
Q. What are the primary metabolic pathways for microbial D-xylonic acid production from D-xylose, and how do they differ in efficiency?
- Methodological Answer : this compound is primarily synthesized via two pathways: (1) the oxidative pathway catalyzed by NAD(P)+-dependent D-xylose dehydrogenase (XylB), producing D-xylonolactone, which is hydrolyzed to this compound , and (2) the Dahms pathway, where D-xylose is oxidized to this compound, followed by dehydration to 2-keto-3-deoxyxylonic acid (2K3DXA) . The oxidative pathway in Gluconobacter oxydans achieves near-theoretical yields (>95%) but requires careful pH control , while the Dahms pathway in engineered Escherichia coli offers flexibility for co-production of glycolic acid or ethylene glycol .
Q. Which microbial strains are most effective for this compound production, and what factors optimize their productivity?
- Methodological Answer : Gluconobacter oxydans is highly efficient due to its membrane-bound glucose dehydrogenase (mGDH), achieving titers up to 588.7 g/L in fed-batch fermentation . Engineered E. coli strains expressing heterologous xylose dehydrogenases (e.g., from Caulobacter crescentus) achieve comparable titers (~200 g/L) but require redox cofactor balancing . Key optimization factors include:
- Overexpression of mGDH or xylose dehydrogenase .
- Fed-batch strategies to mitigate substrate inhibition .
- Tolerance engineering against inhibitors (e.g., furfural) in lignocellulosic hydrolysates .
Advanced Research Questions
Q. How does this compound accumulation impair microbial growth, and how can this be experimentally quantified?
- Methodological Answer : Accumulation acidifies the medium (pH < 4.0), inhibiting cell growth and enzyme activity . Toxicity assays involve:
- Measuring intracellular ATP levels and membrane integrity (e.g., propidium iodide staining) .
- Transcriptomic analysis of stress-response genes (e.g., rpoS, gadA) under acidic conditions .
- Quantifying redox imbalance via NAD+/NADH ratios using enzymatic assays .
Q. What engineering strategies address redox imbalance during this compound synthesis in recombinant strains?
- Methodological Answer : Redox imbalance arises from NADPH depletion in xylose dehydrogenase reactions. Strategies include:
- Co-expression of NADH oxidase (noxE) to regenerate NAD+ .
- Modular cofactor engineering: Overexpression of pyridine nucleotide transhydrogenase (pntAB) to balance NADH/NADPH .
- Co-utilization of glucose and xylose to distribute metabolic flux .
Q. How can conflicting data on the Dahms pathway’s efficiency versus the Weimberg pathway guide strain selection?
- Methodological Answer : The Dahms pathway in C. glutamicum produces pyruvate and glycolaldehyde but requires gluconeogenesis for growth, reducing energy efficiency . The Weimberg pathway (e.g., in Pseudomonas taiwanensis) avoids acid accumulation but has lower xylose uptake rates . Comparative studies should:
- Measure ATP yield and growth rates in minimal media .
- Use 13C metabolic flux analysis to map carbon partitioning .
Methodological and Experimental Design Questions
Q. What fed-batch design parameters maximize this compound titers while minimizing inhibition?
- Methodological Answer :
- Substrate feeding : Maintain xylose concentration <50 g/L to prevent catabolite repression .
- Oxygen supply : Use dissolved oxygen (DO) stat control (20–30%) to enhance mGDH activity in G. oxydans .
- Inhibitor tolerance : Pre-adapt strains to hydrolysates via adaptive laboratory evolution (ALE) .
Q. How can synthetic biology tools dynamically regulate this compound assimilation in response to pH changes?
- Methodological Answer :
- Implement pH-responsive promoters (e.g., PyjhI in E. coli) to activate xylonic acid transporters at low pH .
- Design genetic circuits with CRISPR-dCas9 for tunable expression of lactonase (xylC) to hydrolyze D-xylonolactone .
Q. What analytical methods resolve this compound and its intermediates in complex matrices?
- Methodological Answer :
- Quantification : High-performance liquid chromatography (HPLC) with refractive index detection (RID) or charged aerosol detection (CAD) .
- Structural confirmation : NMR (1H and 13C) for lactone/acid equilibrium analysis .
- Redox profiling : LC-MS/MS for NAD+/NADPH quantification .
Q. Contradictions and Emerging Directions
Q. Why do studies report conflicting efficiencies for photocatalytic vs. microbial this compound production?
- Methodological Answer : Photocatalytic methods (e.g., TiO2/Ti3C2 catalysts) achieve 64.2% yield but require high-energy UV light and lack scalability . Microbial systems offer higher yields (>95%) but face metabolic bottlenecks . Hybrid approaches (e.g., integrating photocatalysis with fermentation) are under exploration .
Q. How can lactonase activity be optimized to prevent D-xylonolactone accumulation in engineered pathways?
Properties
IUPAC Name |
(2R,3S,4R)-2,3,4,5-tetrahydroxypentanoic acid | |
---|---|---|
Source | PubChem | |
URL | https://pubchem.ncbi.nlm.nih.gov | |
Description | Data deposited in or computed by PubChem | |
InChI |
InChI=1S/C5H10O6/c6-1-2(7)3(8)4(9)5(10)11/h2-4,6-9H,1H2,(H,10,11)/t2-,3+,4-/m1/s1 | |
Source | PubChem | |
URL | https://pubchem.ncbi.nlm.nih.gov | |
Description | Data deposited in or computed by PubChem | |
InChI Key |
QXKAIJAYHKCRRA-FLRLBIABSA-N | |
Source | PubChem | |
URL | https://pubchem.ncbi.nlm.nih.gov | |
Description | Data deposited in or computed by PubChem | |
Canonical SMILES |
C(C(C(C(C(=O)O)O)O)O)O | |
Source | PubChem | |
URL | https://pubchem.ncbi.nlm.nih.gov | |
Description | Data deposited in or computed by PubChem | |
Isomeric SMILES |
C([C@H]([C@@H]([C@H](C(=O)O)O)O)O)O | |
Source | PubChem | |
URL | https://pubchem.ncbi.nlm.nih.gov | |
Description | Data deposited in or computed by PubChem | |
Molecular Formula |
C5H10O6 | |
Source | PubChem | |
URL | https://pubchem.ncbi.nlm.nih.gov | |
Description | Data deposited in or computed by PubChem | |
DSSTOX Substance ID |
DTXSID701028292 | |
Record name | D-Xylonic acid | |
Source | EPA DSSTox | |
URL | https://comptox.epa.gov/dashboard/DTXSID701028292 | |
Description | DSSTox provides a high quality public chemistry resource for supporting improved predictive toxicology. | |
Molecular Weight |
166.13 g/mol | |
Source | PubChem | |
URL | https://pubchem.ncbi.nlm.nih.gov | |
Description | Data deposited in or computed by PubChem | |
CAS No. |
526-91-0, 17828-56-7 | |
Record name | D-Xylonic acid | |
Source | CAS Common Chemistry | |
URL | https://commonchemistry.cas.org/detail?cas_rn=526-91-0 | |
Description | CAS Common Chemistry is an open community resource for accessing chemical information. Nearly 500,000 chemical substances from CAS REGISTRY cover areas of community interest, including common and frequently regulated chemicals, and those relevant to high school and undergraduate chemistry classes. This chemical information, curated by our expert scientists, is provided in alignment with our mission as a division of the American Chemical Society. | |
Explanation | The data from CAS Common Chemistry is provided under a CC-BY-NC 4.0 license, unless otherwise stated. | |
Record name | Xylonic acid, D- | |
Source | ChemIDplus | |
URL | https://pubchem.ncbi.nlm.nih.gov/substance/?source=chemidplus&sourceid=0000526910 | |
Description | ChemIDplus is a free, web search system that provides access to the structure and nomenclature authority files used for the identification of chemical substances cited in National Library of Medicine (NLM) databases, including the TOXNET system. | |
Record name | Xylonic acid, DL- | |
Source | ChemIDplus | |
URL | https://pubchem.ncbi.nlm.nih.gov/substance/?source=chemidplus&sourceid=0017828567 | |
Description | ChemIDplus is a free, web search system that provides access to the structure and nomenclature authority files used for the identification of chemical substances cited in National Library of Medicine (NLM) databases, including the TOXNET system. | |
Record name | D-Xylonic acid | |
Source | EPA DSSTox | |
URL | https://comptox.epa.gov/dashboard/DTXSID701028292 | |
Description | DSSTox provides a high quality public chemistry resource for supporting improved predictive toxicology. | |
Record name | XYLONIC ACID, D- | |
Source | FDA Global Substance Registration System (GSRS) | |
URL | https://gsrs.ncats.nih.gov/ginas/app/beta/substances/OCI0V55QO1 | |
Description | The FDA Global Substance Registration System (GSRS) enables the efficient and accurate exchange of information on what substances are in regulated products. Instead of relying on names, which vary across regulatory domains, countries, and regions, the GSRS knowledge base makes it possible for substances to be defined by standardized, scientific descriptions. | |
Explanation | Unless otherwise noted, the contents of the FDA website (www.fda.gov), both text and graphics, are not copyrighted. They are in the public domain and may be republished, reprinted and otherwise used freely by anyone without the need to obtain permission from FDA. Credit to the U.S. Food and Drug Administration as the source is appreciated but not required. | |
Record name | XYLONIC ACID, DL- | |
Source | FDA Global Substance Registration System (GSRS) | |
URL | https://gsrs.ncats.nih.gov/ginas/app/beta/substances/DA38Q87GYH | |
Description | The FDA Global Substance Registration System (GSRS) enables the efficient and accurate exchange of information on what substances are in regulated products. Instead of relying on names, which vary across regulatory domains, countries, and regions, the GSRS knowledge base makes it possible for substances to be defined by standardized, scientific descriptions. | |
Explanation | Unless otherwise noted, the contents of the FDA website (www.fda.gov), both text and graphics, are not copyrighted. They are in the public domain and may be republished, reprinted and otherwise used freely by anyone without the need to obtain permission from FDA. Credit to the U.S. Food and Drug Administration as the source is appreciated but not required. | |
Record name | D-Xylonic acid | |
Source | Human Metabolome Database (HMDB) | |
URL | http://www.hmdb.ca/metabolites/HMDB0059750 | |
Description | The Human Metabolome Database (HMDB) is a freely available electronic database containing detailed information about small molecule metabolites found in the human body. | |
Explanation | HMDB is offered to the public as a freely available resource. Use and re-distribution of the data, in whole or in part, for commercial purposes requires explicit permission of the authors and explicit acknowledgment of the source material (HMDB) and the original publication (see the HMDB citing page). We ask that users who download significant portions of the database cite the HMDB paper in any resulting publications. | |
Retrosynthesis Analysis
AI-Powered Synthesis Planning: Our tool employs the Template_relevance Pistachio, Template_relevance Bkms_metabolic, Template_relevance Pistachio_ringbreaker, Template_relevance Reaxys, Template_relevance Reaxys_biocatalysis model, leveraging a vast database of chemical reactions to predict feasible synthetic routes.
One-Step Synthesis Focus: Specifically designed for one-step synthesis, it provides concise and direct routes for your target compounds, streamlining the synthesis process.
Accurate Predictions: Utilizing the extensive PISTACHIO, BKMS_METABOLIC, PISTACHIO_RINGBREAKER, REAXYS, REAXYS_BIOCATALYSIS database, our tool offers high-accuracy predictions, reflecting the latest in chemical research and data.
Strategy Settings
Precursor scoring | Relevance Heuristic |
---|---|
Min. plausibility | 0.01 |
Model | Template_relevance |
Template Set | Pistachio/Bkms_metabolic/Pistachio_ringbreaker/Reaxys/Reaxys_biocatalysis |
Top-N result to add to graph | 6 |
Feasible Synthetic Routes
Disclaimer and Information on In-Vitro Research Products
Please be aware that all articles and product information presented on BenchChem are intended solely for informational purposes. The products available for purchase on BenchChem are specifically designed for in-vitro studies, which are conducted outside of living organisms. In-vitro studies, derived from the Latin term "in glass," involve experiments performed in controlled laboratory settings using cells or tissues. It is important to note that these products are not categorized as medicines or drugs, and they have not received approval from the FDA for the prevention, treatment, or cure of any medical condition, ailment, or disease. We must emphasize that any form of bodily introduction of these products into humans or animals is strictly prohibited by law. It is essential to adhere to these guidelines to ensure compliance with legal and ethical standards in research and experimentation.