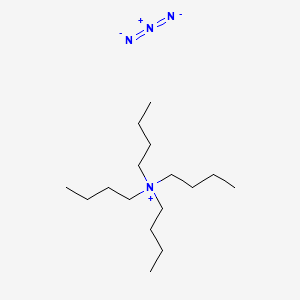
Tetrabutylammonium azide
Overview
Description
Tetrabutylammonium azide, also known as this compound, is a useful research compound. Its molecular formula is C16H36N4 and its molecular weight is 284.48 g/mol. The purity is usually 95%.
The exact mass of the compound this compound is unknown and the complexity rating of the compound is unknown. The United Nations designated GHS hazard class pictogram is Acute Toxic;Irritant;Environmental Hazard, and the GHS signal word is DangerThe storage condition is unknown. Please store according to label instructions upon receipt of goods.
BenchChem offers high-quality this compound suitable for many research applications. Different packaging options are available to accommodate customers' requirements. Please inquire for more information about this compound including the price, delivery time, and more detailed information at info@benchchem.com.
Scientific Research Applications
Anionic Polymerization
Tetrabutylammonium azide has been found effective in initiating the anionic polymerization of ethyl acrylate, resulting in azide-end polymers with narrow molecular weight distributions. These polymers are highly reactive and suitable for copper-catalyzed azide–alkyne cycloaddition (Kataoka et al., 2017).
Ring-Opening Polymerization
This compound serves as an initiator for anionic ring-opening polymerization, particularly for synthesizing azide-terminated linear poly(glycidyl phenyl ether) and generating pure cyclic structures. This process is vital in achieving high end-group fidelity in polymers (Ochs et al., 2018).
Catalysis in Organic Synthesis
The compound is used in the efficient azidation of 1,3-dicarbonyl compounds, resulting in tertiary azides with good yields. This method is practical and green, providing a variety of azidated keto esters, amides, and ketones (Dhineshkumar & Prabhu, 2016).
Pharmaceutical Applications
In pharmaceutical research, this compound finds use in HPLC analysis, particularly as a phase-transfer agent in PET radiochemistry. Its analysis is crucial due to its toxicity (Bogni et al., 2019).
Photocatalysis
Tetrabutylammonium derivatives are used in photocatalytic activities, such as the activation of Si−H bonds in silyl hydrides for hydrosilylation of electron‐poor alkenes. This process depends on the type of silyl hydride used (Qrareya et al., 2015).
Synthesis of Functionalized Molecules
It is instrumental in synthesizing highly functionalized cyclopentenes, offering a facile route to multifunctionalized molecules that can serve as precursors for further chemical synthesis (Alishetty et al., 2018).
Mechanism of Action
Target of Action
Tetrabutylammonium azide is a versatile reagent used in organic synthesis . It primarily targets organic molecules, facilitating various chemical transformations. It is particularly effective in the synthesis of heteroarylannulated bicyclic morpholines and cyanimide-based inhibitors of cathepsin C .
Mode of Action
This compound acts as a catalyst and a reagent in several chemical reactions . It facilitates the aerobic oxidative transformation of primary azides to nitriles and substitution reactions at tetracoordinate boron . As a catalyst, it enhances the reaction rate and product yield without being consumed in the process .
Biochemical Pathways
The exact biochemical pathways influenced by this compound are dependent on the specific reactions it catalyzes. For instance, in the synthesis of heteroarylannulated bicyclic morpholines, it may influence the pathways related to the formation of these complex structures .
Pharmacokinetics
Its solubility in both aqueous and organic solvents suggests it may have good bioavailability .
Result of Action
The result of this compound’s action is the successful synthesis of various organic compounds. For example, it can help produce heteroarylannulated bicyclic morpholines and cyanimide-based inhibitors of cathepsin C . It also aids in the aerobic oxidative transformation of primary azides to nitriles .
Action Environment
The action of this compound can be influenced by environmental factors such as temperature, pH, and the presence of other reagents. For instance, it is typically stored at temperatures between 2-8°C to maintain its stability . The presence of other reagents can also affect its efficacy as a catalyst or reagent .
Safety and Hazards
Tetrabutylammonium azide is toxic if swallowed, in contact with skin, or if inhaled . It causes skin irritation and serious eye irritation . It is a flammable solid . It is very toxic to aquatic life with long-lasting effects . It is recommended to avoid breathing dust, fume, gas, mist, vapors, spray, and to avoid contact with skin and eyes .
Biochemical Analysis
Biochemical Properties
Tetrabutylammonium azide plays a significant role in biochemical reactions, particularly in the synthesis of heteroarylannulated bicyclic morpholines and cyanimide-based inhibitors of cathepsin C . It interacts with various enzymes and proteins, facilitating the aerobic oxidative transformation of primary azides to nitriles and substitution reactions at tetracoordinate boron . These interactions are crucial for the formation of cyclic carbonates and other complex organic molecules.
Cellular Effects
This compound influences various cellular processes, including cell signaling pathways, gene expression, and cellular metabolism. It has been observed to affect the respiratory system and cause skin and eye irritation . The compound’s impact on cell function is primarily due to its ability to interact with cellular proteins and enzymes, altering their activity and leading to changes in cellular behavior.
Molecular Mechanism
At the molecular level, this compound exerts its effects through binding interactions with biomolecules. It acts as a catalyst in various biochemical reactions, including the aerobic oxidative transformation of primary azides to nitriles . The compound’s ability to inhibit or activate enzymes is a key aspect of its mechanism of action, leading to changes in gene expression and cellular function.
Temporal Effects in Laboratory Settings
In laboratory settings, the effects of this compound can change over time. The compound is stable under specific storage conditions (2-8°C) and has a melting point of 84-88°C . Long-term exposure to this compound can lead to chronic effects on cellular function, including potential damage to the respiratory system . Its stability and degradation over time are important factors to consider in experimental setups.
Dosage Effects in Animal Models
The effects of this compound vary with different dosages in animal models. At lower doses, the compound may facilitate specific biochemical reactions without causing significant adverse effects. At higher doses, this compound can exhibit toxic effects, including acute toxicity and potential damage to organs . Understanding the dosage thresholds is crucial for safe and effective use in research.
Metabolic Pathways
This compound is involved in various metabolic pathways, interacting with enzymes and cofactors to facilitate biochemical reactions. It plays a role in the nucleotide salvage pathway, where azide-modified nucleosides are converted to nucleotide triphosphates . These interactions can affect metabolic flux and metabolite levels, influencing overall cellular metabolism.
Transport and Distribution
Within cells and tissues, this compound is transported and distributed through interactions with transporters and binding proteins. Its localization and accumulation can impact its activity and function, influencing the outcomes of biochemical reactions . Understanding these transport mechanisms is essential for optimizing the compound’s use in research.
Subcellular Localization
This compound’s subcellular localization is determined by targeting signals and post-translational modifications that direct it to specific compartments or organelles. Its activity and function can be influenced by its localization within the cell, affecting the efficiency of biochemical reactions . Mapping its subcellular distribution is important for understanding its role in cellular processes.
Properties
IUPAC Name |
tetrabutylazanium;azide | |
---|---|---|
Source | PubChem | |
URL | https://pubchem.ncbi.nlm.nih.gov | |
Description | Data deposited in or computed by PubChem | |
InChI |
InChI=1S/C16H36N.N3/c1-5-9-13-17(14-10-6-2,15-11-7-3)16-12-8-4;1-3-2/h5-16H2,1-4H3;/q+1;-1 | |
Source | PubChem | |
URL | https://pubchem.ncbi.nlm.nih.gov | |
Description | Data deposited in or computed by PubChem | |
InChI Key |
GMRIOAVKKGNMMV-UHFFFAOYSA-N | |
Source | PubChem | |
URL | https://pubchem.ncbi.nlm.nih.gov | |
Description | Data deposited in or computed by PubChem | |
Canonical SMILES |
CCCC[N+](CCCC)(CCCC)CCCC.[N-]=[N+]=[N-] | |
Source | PubChem | |
URL | https://pubchem.ncbi.nlm.nih.gov | |
Description | Data deposited in or computed by PubChem | |
Molecular Formula |
C16H36N4 | |
Source | PubChem | |
URL | https://pubchem.ncbi.nlm.nih.gov | |
Description | Data deposited in or computed by PubChem | |
Related CAS |
10549-76-5 (Parent) | |
Record name | 1-Butanaminium, N,N,N-tributyl-, azide (1:1) | |
Source | ChemIDplus | |
URL | https://pubchem.ncbi.nlm.nih.gov/substance/?source=chemidplus&sourceid=0000993226 | |
Description | ChemIDplus is a free, web search system that provides access to the structure and nomenclature authority files used for the identification of chemical substances cited in National Library of Medicine (NLM) databases, including the TOXNET system. | |
DSSTOX Substance ID |
DTXSID1061360 | |
Record name | 1-Butanaminium, N,N,N-tributyl-, azide | |
Source | EPA DSSTox | |
URL | https://comptox.epa.gov/dashboard/DTXSID1061360 | |
Description | DSSTox provides a high quality public chemistry resource for supporting improved predictive toxicology. | |
Molecular Weight |
284.48 g/mol | |
Source | PubChem | |
URL | https://pubchem.ncbi.nlm.nih.gov | |
Description | Data deposited in or computed by PubChem | |
CAS No. |
993-22-6 | |
Record name | 1-Butanaminium, N,N,N-tributyl-, azide (1:1) | |
Source | CAS Common Chemistry | |
URL | https://commonchemistry.cas.org/detail?cas_rn=993-22-6 | |
Description | CAS Common Chemistry is an open community resource for accessing chemical information. Nearly 500,000 chemical substances from CAS REGISTRY cover areas of community interest, including common and frequently regulated chemicals, and those relevant to high school and undergraduate chemistry classes. This chemical information, curated by our expert scientists, is provided in alignment with our mission as a division of the American Chemical Society. | |
Explanation | The data from CAS Common Chemistry is provided under a CC-BY-NC 4.0 license, unless otherwise stated. | |
Record name | 1-Butanaminium, N,N,N-tributyl-, azide (1:1) | |
Source | ChemIDplus | |
URL | https://pubchem.ncbi.nlm.nih.gov/substance/?source=chemidplus&sourceid=0000993226 | |
Description | ChemIDplus is a free, web search system that provides access to the structure and nomenclature authority files used for the identification of chemical substances cited in National Library of Medicine (NLM) databases, including the TOXNET system. | |
Record name | 1-Butanaminium, N,N,N-tributyl-, azide (1:1) | |
Source | EPA Chemicals under the TSCA | |
URL | https://www.epa.gov/chemicals-under-tsca | |
Description | EPA Chemicals under the Toxic Substances Control Act (TSCA) collection contains information on chemicals and their regulations under TSCA, including non-confidential content from the TSCA Chemical Substance Inventory and Chemical Data Reporting. | |
Record name | 1-Butanaminium, N,N,N-tributyl-, azide | |
Source | EPA DSSTox | |
URL | https://comptox.epa.gov/dashboard/DTXSID1061360 | |
Description | DSSTox provides a high quality public chemistry resource for supporting improved predictive toxicology. | |
Record name | 1-Butanaminium, N,N,N-tributyl-, azide (1:1) | |
Source | European Chemicals Agency (ECHA) | |
URL | https://echa.europa.eu/substance-information/-/substanceinfo/100.120.827 | |
Description | The European Chemicals Agency (ECHA) is an agency of the European Union which is the driving force among regulatory authorities in implementing the EU's groundbreaking chemicals legislation for the benefit of human health and the environment as well as for innovation and competitiveness. | |
Explanation | Use of the information, documents and data from the ECHA website is subject to the terms and conditions of this Legal Notice, and subject to other binding limitations provided for under applicable law, the information, documents and data made available on the ECHA website may be reproduced, distributed and/or used, totally or in part, for non-commercial purposes provided that ECHA is acknowledged as the source: "Source: European Chemicals Agency, http://echa.europa.eu/". Such acknowledgement must be included in each copy of the material. ECHA permits and encourages organisations and individuals to create links to the ECHA website under the following cumulative conditions: Links can only be made to webpages that provide a link to the Legal Notice page. | |
Retrosynthesis Analysis
AI-Powered Synthesis Planning: Our tool employs the Template_relevance Pistachio, Template_relevance Bkms_metabolic, Template_relevance Pistachio_ringbreaker, Template_relevance Reaxys, Template_relevance Reaxys_biocatalysis model, leveraging a vast database of chemical reactions to predict feasible synthetic routes.
One-Step Synthesis Focus: Specifically designed for one-step synthesis, it provides concise and direct routes for your target compounds, streamlining the synthesis process.
Accurate Predictions: Utilizing the extensive PISTACHIO, BKMS_METABOLIC, PISTACHIO_RINGBREAKER, REAXYS, REAXYS_BIOCATALYSIS database, our tool offers high-accuracy predictions, reflecting the latest in chemical research and data.
Strategy Settings
Precursor scoring | Relevance Heuristic |
---|---|
Min. plausibility | 0.01 |
Model | Template_relevance |
Template Set | Pistachio/Bkms_metabolic/Pistachio_ringbreaker/Reaxys/Reaxys_biocatalysis |
Top-N result to add to graph | 6 |
Feasible Synthetic Routes
Q1: What is the primary use of tetrabutylammonium azide in scientific research?
A1: this compound (TBAN3) is widely employed as a reagent for introducing azide groups into molecules. [, , , , ] This is a crucial step in various chemical syntheses, particularly in preparing polymers with tailored functionalities and synthesizing pharmaceutical compounds. [, , , , ]
Q2: How does the structure of TBAN3 contribute to its reactivity?
A2: TBAN3 consists of a tetrabutylammonium cation ([(CH3CH2CH2CH2)4N]+) and an azide anion (N3−). [] The bulky, non-polar tetrabutylammonium cation enhances the solubility of the azide anion in organic solvents, [, ] while also increasing its nucleophilicity, making it more reactive towards electrophilic substrates. [, , ]
Q3: Can you provide examples of reactions where TBAN3 acts as a nucleophile?
A3: TBAN3 readily reacts with alkyl halides, replacing the halogen atom with an azide group. This reaction is particularly effective with tertiary and secondary halides, allowing the synthesis of alkyl azides. [] This reactivity is utilized in end-group transformations of halide-end polymethacrylates, converting them into azido-end polymers for various applications. []
Q4: Are there any specific advantages of using TBAN3 for azidation compared to other azide sources?
A4: Compared to inorganic azide salts like sodium azide, TBAN3 exhibits superior solubility in organic solvents. [, , , ] This enhanced solubility allows for faster and more efficient reactions, often requiring a lower excess of TBAN3. [, ] Additionally, its use can enable metal-free synthesis routes, which can be beneficial for synthesizing biologically active compounds. [, ]
Q5: What role does TBAN3 play in the synthesis of cyclic poly(glycidyl phenyl ether)?
A5: TBAN3 can initiate the anionic ring-opening polymerization of glycidyl phenyl ether, producing linear poly(glycidyl phenyl ether) with an azide group at one end. [] This azide-terminated polymer can then undergo a copper-catalyzed alkyne–azide cycloaddition (“click” reaction), leading to the formation of monocyclic poly(glycidyl phenyl ether) with high purity. []
Q6: How is TBAN3 used in the synthesis of "clickable" polymers?
A6: TBAN3 is crucial for introducing azide functionalities at the end of polymer chains. [, ] For instance, α-azido, ω-hydroxy heterofunctional polyethylene oxide (PEO) can be prepared by anionic ring-opening polymerization using TBAN3 as an initiator. [] This functionalized PEO can then be further modified for applications like AGET ATRP (activators generated by electron transfer for atom transfer radical polymerization), leading to the creation of star-shaped PEO with clickable azide groups. []
Q7: Can you provide an example of TBAN3 being used in the synthesis of a specific class of organic compounds?
A7: TBAN3 is a crucial reagent in synthesizing 5-substituted 1H-tetrazoles. [] It acts as both the azide source and, upon reaction with the reaction solvent tetrabutylammonium bromide, generates this compound in situ, which is the active nucleophile in the reaction. []
Q8: What is the role of TBAN3 in studying the reactivity of vinyl cations?
A8: TBAN3 can act as a nucleophile to trap vinyl cations generated during photolysis reactions. [, ] For example, in the photolysis of vinyl bromides, TBAN3 effectively traps the transient vinyl cation, forming the corresponding vinyl azide. [, ] This reaction provides valuable insights into the reactivity and lifetime of vinyl cations.
Q9: Has TBAN3 been used in the synthesis of radiolabeled compounds for medical imaging?
A9: Yes, TBAN3 has been employed in the synthesis of [11C]physostigmine, a radiolabeled inhibitor of acetylcholinesterase. [] This radiolabeled compound is used in positron emission tomography (PET) to study acetylcholinesterase activity in the brain. []
Disclaimer and Information on In-Vitro Research Products
Please be aware that all articles and product information presented on BenchChem are intended solely for informational purposes. The products available for purchase on BenchChem are specifically designed for in-vitro studies, which are conducted outside of living organisms. In-vitro studies, derived from the Latin term "in glass," involve experiments performed in controlled laboratory settings using cells or tissues. It is important to note that these products are not categorized as medicines or drugs, and they have not received approval from the FDA for the prevention, treatment, or cure of any medical condition, ailment, or disease. We must emphasize that any form of bodily introduction of these products into humans or animals is strictly prohibited by law. It is essential to adhere to these guidelines to ensure compliance with legal and ethical standards in research and experimentation.