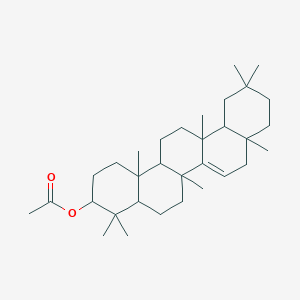
Taraxeryl acetate
Overview
Description
Taraxeryl acetate, also known as acetyl taraxerol, is a naturally occurring pentacyclic triterpenoid compound. It is derived from taraxerol, which is found in various plant species, including dandelions. This compound has garnered significant attention due to its diverse biological activities and potential therapeutic applications .
Preparation Methods
Synthetic Routes and Reaction Conditions
Taraxeryl acetate can be synthesized through the acetylation of taraxerol. The process typically involves the reaction of taraxerol with acetic anhydride in the presence of a catalyst such as pyridine. The reaction is carried out under reflux conditions, and the product is purified through recrystallization .
Industrial Production Methods
Industrial production of this compound often involves the extraction of taraxerol from plant sources, followed by its acetylation. The extraction process may include solvent extraction, ultrasonic extraction, or supercritical fluid extraction. The acetylation step is similar to the laboratory synthesis, involving acetic anhydride and a catalyst .
Chemical Reactions Analysis
Types of Reactions
Taraxeryl acetate undergoes various chemical reactions, including:
Oxidation: this compound can be oxidized to form corresponding ketones or carboxylic acids.
Reduction: Reduction reactions can convert this compound back to taraxerol.
Substitution: Substitution reactions can occur at the acetate group, leading to the formation of different derivatives.
Common Reagents and Conditions
Oxidation: Common oxidizing agents include potassium permanganate and chromium trioxide.
Reduction: Reducing agents such as lithium aluminum hydride or sodium borohydride are used.
Substitution: Various nucleophiles can be used for substitution reactions, depending on the desired product.
Major Products
Scientific Research Applications
Chemistry: It serves as a precursor for the synthesis of other triterpenoid derivatives.
Biology: Taraxeryl acetate exhibits cytotoxic effects against certain cancer cell lines, making it a candidate for anticancer research.
Medicine: It has shown anti-inflammatory, antioxidant, and antimicrobial properties, suggesting potential therapeutic uses.
Industry: The compound is used in the development of natural product-based pharmaceuticals and cosmetics
Mechanism of Action
The mechanism of action of taraxeryl acetate involves multiple molecular targets and pathways:
Cytotoxic Effects: It induces apoptosis in cancer cells by activating caspases and promoting cell cycle arrest.
Anti-inflammatory Activity: this compound inhibits the production of pro-inflammatory cytokines and reduces oxidative stress.
Antimicrobial Activity: It disrupts microbial cell membranes and inhibits essential enzymes
Comparison with Similar Compounds
Taraxeryl acetate is unique among similar compounds due to its specific biological activities and structural features. Similar compounds include:
Taraxerol: The parent compound, which lacks the acetyl group.
Taraxerone: An oxidized derivative of taraxerol.
Epitaraxerol: A stereoisomer of taraxerol.
Myricadiol: Another triterpenoid with similar structural features
Properties
IUPAC Name |
(4,4,6a,6a,8a,11,11,14b-octamethyl-1,2,3,4a,5,6,8,9,10,12,12a,13,14,14a-tetradecahydropicen-3-yl) acetate | |
---|---|---|
Details | Computed by LexiChem 2.6.6 (PubChem release 2019.06.18) | |
Source | PubChem | |
URL | https://pubchem.ncbi.nlm.nih.gov | |
Description | Data deposited in or computed by PubChem | |
InChI |
InChI=1S/C32H52O2/c1-21(33)34-26-13-17-30(7)22(28(26,4)5)11-15-31(8)23-10-14-29(6)19-18-27(2,3)20-25(29)32(23,9)16-12-24(30)31/h10,22,24-26H,11-20H2,1-9H3 | |
Details | Computed by InChI 1.0.5 (PubChem release 2019.06.18) | |
Source | PubChem | |
URL | https://pubchem.ncbi.nlm.nih.gov | |
Description | Data deposited in or computed by PubChem | |
InChI Key |
YWJGYBXHXATAQY-UHFFFAOYSA-N | |
Details | Computed by InChI 1.0.5 (PubChem release 2019.06.18) | |
Source | PubChem | |
URL | https://pubchem.ncbi.nlm.nih.gov | |
Description | Data deposited in or computed by PubChem | |
Canonical SMILES |
CC(=O)OC1CCC2(C(C1(C)C)CCC3(C2CCC4(C3=CCC5(C4CC(CC5)(C)C)C)C)C)C | |
Details | Computed by OEChem 2.1.5 (PubChem release 2019.06.18) | |
Source | PubChem | |
URL | https://pubchem.ncbi.nlm.nih.gov | |
Description | Data deposited in or computed by PubChem | |
Molecular Formula |
C32H52O2 | |
Details | Computed by PubChem 2.1 (PubChem release 2019.06.18) | |
Source | PubChem | |
URL | https://pubchem.ncbi.nlm.nih.gov | |
Description | Data deposited in or computed by PubChem | |
Molecular Weight |
468.8 g/mol | |
Details | Computed by PubChem 2.1 (PubChem release 2021.05.07) | |
Source | PubChem | |
URL | https://pubchem.ncbi.nlm.nih.gov | |
Description | Data deposited in or computed by PubChem | |
Retrosynthesis Analysis
AI-Powered Synthesis Planning: Our tool employs the Template_relevance Pistachio, Template_relevance Bkms_metabolic, Template_relevance Pistachio_ringbreaker, Template_relevance Reaxys, Template_relevance Reaxys_biocatalysis model, leveraging a vast database of chemical reactions to predict feasible synthetic routes.
One-Step Synthesis Focus: Specifically designed for one-step synthesis, it provides concise and direct routes for your target compounds, streamlining the synthesis process.
Accurate Predictions: Utilizing the extensive PISTACHIO, BKMS_METABOLIC, PISTACHIO_RINGBREAKER, REAXYS, REAXYS_BIOCATALYSIS database, our tool offers high-accuracy predictions, reflecting the latest in chemical research and data.
Strategy Settings
Precursor scoring | Relevance Heuristic |
---|---|
Min. plausibility | 0.01 |
Model | Template_relevance |
Template Set | Pistachio/Bkms_metabolic/Pistachio_ringbreaker/Reaxys/Reaxys_biocatalysis |
Top-N result to add to graph | 6 |
Feasible Synthetic Routes
Disclaimer and Information on In-Vitro Research Products
Please be aware that all articles and product information presented on BenchChem are intended solely for informational purposes. The products available for purchase on BenchChem are specifically designed for in-vitro studies, which are conducted outside of living organisms. In-vitro studies, derived from the Latin term "in glass," involve experiments performed in controlled laboratory settings using cells or tissues. It is important to note that these products are not categorized as medicines or drugs, and they have not received approval from the FDA for the prevention, treatment, or cure of any medical condition, ailment, or disease. We must emphasize that any form of bodily introduction of these products into humans or animals is strictly prohibited by law. It is essential to adhere to these guidelines to ensure compliance with legal and ethical standards in research and experimentation.