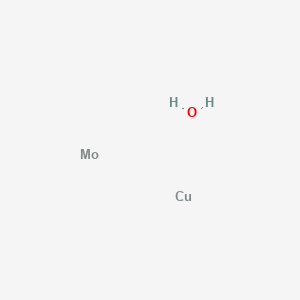
Copper;molybdenum;hydrate
- Click on QUICK INQUIRY to receive a quote from our team of experts.
- With the quality product at a COMPETITIVE price, you can focus more on your research.
Overview
Description
Copper;molybdenum;hydrate is a compound that combines copper, molybdenum, and water molecules. This compound is of significant interest due to its unique chemical properties and potential applications in various fields, including catalysis, materials science, and electrochemistry. The presence of both copper and molybdenum in the compound allows for a diverse range of chemical behaviors and interactions.
Preparation Methods
Synthetic Routes and Reaction Conditions
The preparation of copper;molybdenum;hydrate typically involves the reaction of copper salts with molybdenum compounds in an aqueous medium. One common method is the co-precipitation technique, where copper(II) sulfate and ammonium molybdate are dissolved in water, followed by the addition of a precipitating agent such as sodium hydroxide. The resulting precipitate is then filtered, washed, and dried to obtain the hydrated compound.
Industrial Production Methods
Industrial production of this compound often involves the roasting of molybdenite (MoS₂) concentrates with copper-containing materials. The roasted product is then subjected to hydrometallurgical processes, such as leaching with acids or bases, to extract the desired compound. This method allows for the efficient recovery of both copper and molybdenum from their respective ores.
Chemical Reactions Analysis
Types of Reactions
Copper;molybdenum;hydrate undergoes various chemical reactions, including oxidation, reduction, and substitution reactions. For instance, the compound can be oxidized to form higher oxidation state species, or reduced to yield lower oxidation state products.
Common Reagents and Conditions
Oxidation: The compound can be oxidized using oxidizing agents such as hydrogen peroxide or potassium permanganate under acidic conditions.
Reduction: Reducing agents like hydrogen gas or sodium borohydride can be used to reduce the compound under controlled conditions.
Substitution: Substitution reactions can occur in the presence of ligands such as ammonia or ethylenediamine, leading to the formation of new coordination complexes.
Major Products Formed
The major products formed from these reactions depend on the specific conditions and reagents used. For example, oxidation may yield copper(II) oxide and molybdenum trioxide, while reduction could produce copper metal and molybdenum dioxide.
Scientific Research Applications
Copper;molybdenum;hydrate has a wide range of scientific research applications:
Chemistry: It is used as a catalyst in various chemical reactions, including hydrogen evolution reactions and oxidation-reduction processes.
Biology: The compound is studied for its potential antimicrobial properties and its role in biological systems involving copper and molybdenum enzymes.
Medicine: Research is ongoing to explore its potential use in medical applications, such as drug delivery systems and diagnostic agents.
Industry: It is utilized in the production of advanced materials, including high-strength alloys and corrosion-resistant coatings.
Mechanism of Action
The mechanism of action of copper;molybdenum;hydrate involves the interaction of copper and molybdenum ions with various molecular targets. In catalytic processes, the compound facilitates electron transfer reactions, often involving the formation and breaking of chemical bonds. The presence of water molecules in the hydrate form can also influence the reactivity and stability of the compound.
Comparison with Similar Compounds
Similar Compounds
Copper(II) sulfate pentahydrate (CuSO₄·5H₂O): A well-known copper compound with similar hydration properties.
Ammonium molybdate tetrahydrate ((NH₄)₆Mo₇O₂₄·4H₂O): A molybdenum compound with comparable hydration characteristics.
Molybdenum trioxide (MoO₃): An oxide form of molybdenum with distinct chemical properties.
Uniqueness
Copper;molybdenum;hydrate is unique due to the combination of copper and molybdenum in a single compound, which allows for a diverse range of chemical behaviors and applications. The presence of both metals can enhance the catalytic activity and provide synergistic effects that are not observed in the individual components.
Properties
Molecular Formula |
CuH2MoO |
---|---|
Molecular Weight |
177.51 g/mol |
IUPAC Name |
copper;molybdenum;hydrate |
InChI |
InChI=1S/Cu.Mo.H2O/h;;1H2 |
InChI Key |
UXYZUAHIFMIZRN-UHFFFAOYSA-N |
Canonical SMILES |
O.[Cu].[Mo] |
Origin of Product |
United States |
Disclaimer and Information on In-Vitro Research Products
Please be aware that all articles and product information presented on BenchChem are intended solely for informational purposes. The products available for purchase on BenchChem are specifically designed for in-vitro studies, which are conducted outside of living organisms. In-vitro studies, derived from the Latin term "in glass," involve experiments performed in controlled laboratory settings using cells or tissues. It is important to note that these products are not categorized as medicines or drugs, and they have not received approval from the FDA for the prevention, treatment, or cure of any medical condition, ailment, or disease. We must emphasize that any form of bodily introduction of these products into humans or animals is strictly prohibited by law. It is essential to adhere to these guidelines to ensure compliance with legal and ethical standards in research and experimentation.