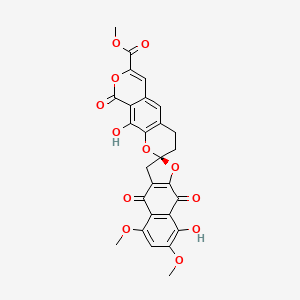
beta-Rubromycin
Overview
Description
Beta-Rubromycin is a quinone antibiotic agent . It is found to inhibit reverse HIV-1 RT (transcriptase from HIV-1) to a greater degree than it inhibits cellular DNA polymerase α .
Molecular Structure Analysis
Beta-Rubromycin contains a total of 64 bonds; 44 non-H bonds, 18 multiple bonds, 4 rotatable bonds, 6 double bonds, 12 aromatic bonds, 1 five-membered ring, 5 six-membered rings, 1 nine-membered ring, 3 ten-membered rings, 1 aliphatic ester, 1 aromatic ester, 2 aromatic ketones, and 2 aromatic hydroxyls .
Chemical Reactions Analysis
The rubromycins are an ever-growing family of natural products isolated from various Actinomycetes over the last 60 years . They exhibit a highly attractive array of antimicrobial and enzyme activity .
Physical And Chemical Properties Analysis
Beta-Rubromycin has a molecular weight of 536.44 and a chemical formula of C27H20O12 .
Scientific Research Applications
Anticancer Properties
β-rubromycin exhibits potent cytotoxic activity against cancer cells. Researchers have found that it can inhibit cancer cell growth, making it a promising candidate for cancer therapy . Further studies explore its mechanisms of action and potential synergies with other anticancer agents.
Chemical Genetics
In the study of life-stage transitions in Phytophthora infestans (a plant pathogen causing late blight in potatoes and tomatoes), researchers have used β-rubromycin as a chemical genetic tool. By screening for stage-specific inhibitors, they aim to understand the molecular mechanisms underlying these transitions .
Polyketide Biosynthesis
β-rubromycin is involved in polyketide biosynthesis pathways. A dedicated acetyltransferase modifies a redox-tailoring enzyme, enabling pathway furcation and alternating pharmacophore assembly in rubromycin biosynthesis . This intricate process contributes to the compound’s unique structure and properties.
Mechanism of Action
Safety and Hazards
Future Directions
properties
IUPAC Name |
methyl (2S)-8',10-dihydroxy-5',7'-dimethoxy-4',9,9'-trioxospiro[3,4-dihydropyrano[4,3-g]chromene-2,2'-3H-benzo[f][1]benzofuran]-7-carboxylate | |
---|---|---|
Source | PubChem | |
URL | https://pubchem.ncbi.nlm.nih.gov | |
Description | Data deposited in or computed by PubChem | |
InChI |
InChI=1S/C27H20O12/c1-34-13-8-14(35-2)20(29)18-17(13)19(28)12-9-27(39-24(12)22(18)31)5-4-10-6-11-7-15(25(32)36-3)37-26(33)16(11)21(30)23(10)38-27/h6-8,29-30H,4-5,9H2,1-3H3/t27-/m0/s1 | |
Source | PubChem | |
URL | https://pubchem.ncbi.nlm.nih.gov | |
Description | Data deposited in or computed by PubChem | |
InChI Key |
FXCBZGHGMRSWJD-MHZLTWQESA-N | |
Source | PubChem | |
URL | https://pubchem.ncbi.nlm.nih.gov | |
Description | Data deposited in or computed by PubChem | |
Canonical SMILES |
COC1=CC(=C(C2=C1C(=O)C3=C(C2=O)OC4(C3)CCC5=C(O4)C(=C6C(=C5)C=C(OC6=O)C(=O)OC)O)O)OC | |
Source | PubChem | |
URL | https://pubchem.ncbi.nlm.nih.gov | |
Description | Data deposited in or computed by PubChem | |
Isomeric SMILES |
COC1=CC(=C(C2=C1C(=O)C3=C(C2=O)O[C@]4(C3)CCC5=C(O4)C(=C6C(=C5)C=C(OC6=O)C(=O)OC)O)O)OC | |
Source | PubChem | |
URL | https://pubchem.ncbi.nlm.nih.gov | |
Description | Data deposited in or computed by PubChem | |
Molecular Formula |
C27H20O12 | |
Source | PubChem | |
URL | https://pubchem.ncbi.nlm.nih.gov | |
Description | Data deposited in or computed by PubChem | |
DSSTOX Substance ID |
DTXSID30181715 | |
Record name | beta-Rubromycin | |
Source | EPA DSSTox | |
URL | https://comptox.epa.gov/dashboard/DTXSID30181715 | |
Description | DSSTox provides a high quality public chemistry resource for supporting improved predictive toxicology. | |
Molecular Weight |
536.4 g/mol | |
Source | PubChem | |
URL | https://pubchem.ncbi.nlm.nih.gov | |
Description | Data deposited in or computed by PubChem | |
Product Name |
beta-Rubromycin | |
CAS RN |
27267-70-5 | |
Record name | beta-Rubromycin | |
Source | ChemIDplus | |
URL | https://pubchem.ncbi.nlm.nih.gov/substance/?source=chemidplus&sourceid=0027267705 | |
Description | ChemIDplus is a free, web search system that provides access to the structure and nomenclature authority files used for the identification of chemical substances cited in National Library of Medicine (NLM) databases, including the TOXNET system. | |
Record name | beta-Rubromycin | |
Source | EPA DSSTox | |
URL | https://comptox.epa.gov/dashboard/DTXSID30181715 | |
Description | DSSTox provides a high quality public chemistry resource for supporting improved predictive toxicology. | |
Retrosynthesis Analysis
AI-Powered Synthesis Planning: Our tool employs the Template_relevance Pistachio, Template_relevance Bkms_metabolic, Template_relevance Pistachio_ringbreaker, Template_relevance Reaxys, Template_relevance Reaxys_biocatalysis model, leveraging a vast database of chemical reactions to predict feasible synthetic routes.
One-Step Synthesis Focus: Specifically designed for one-step synthesis, it provides concise and direct routes for your target compounds, streamlining the synthesis process.
Accurate Predictions: Utilizing the extensive PISTACHIO, BKMS_METABOLIC, PISTACHIO_RINGBREAKER, REAXYS, REAXYS_BIOCATALYSIS database, our tool offers high-accuracy predictions, reflecting the latest in chemical research and data.
Strategy Settings
Precursor scoring | Relevance Heuristic |
---|---|
Min. plausibility | 0.01 |
Model | Template_relevance |
Template Set | Pistachio/Bkms_metabolic/Pistachio_ringbreaker/Reaxys/Reaxys_biocatalysis |
Top-N result to add to graph | 6 |
Feasible Synthetic Routes
Q & A
Q1: What is the primary mechanism of action of beta-rubromycin?
A1: Beta-rubromycin acts as a potent inhibitor of human telomerase []. It accomplishes this through competitive interaction with the telomerase substrate primer, essentially blocking the enzyme's ability to elongate telomeres []. This inhibition was demonstrated in vitro using a modified telomeric repeat amplification protocol (TRAP) assay [].
Q2: How does the structure of beta-rubromycin contribute to its inhibitory activity?
A2: The spiroketal system, formed by the benzofuran and benzodipyran rings, is crucial for beta-rubromycin's telomerase inhibition []. This was confirmed by comparing its activity to alpha-rubromycin, which lacks the intact spiroketal system and shows significantly reduced potency []. Further research suggests that beta-rubromycin might bind to the active site of its target enzymes, possibly competing with nucleotide substrates [].
Q3: Has the synthesis of beta-rubromycin and its analogs been explored?
A4: Yes, researchers have developed a facile synthesis method for naphthoquinone spiroketals, including beta-rubromycin and its analogs, using a diastereoselective oxidative [3 + 2] cycloaddition of chiral enol ethers and hydroxynaphthoquinone []. This strategy allows for the enantioselective synthesis of these compounds, opening avenues for further research into their structure-activity relationships and therapeutic potential [].
Q4: Are there any concerns regarding the toxicity of beta-rubromycin?
A5: While beta-rubromycin demonstrates promising inhibitory activity against cancer cell proliferation in vitro, it also exhibits non-specific cytotoxicity []. Further research is necessary to explore its safety profile, potential for targeted delivery, and to develop analogs with improved selectivity and reduced toxicity.
Disclaimer and Information on In-Vitro Research Products
Please be aware that all articles and product information presented on BenchChem are intended solely for informational purposes. The products available for purchase on BenchChem are specifically designed for in-vitro studies, which are conducted outside of living organisms. In-vitro studies, derived from the Latin term "in glass," involve experiments performed in controlled laboratory settings using cells or tissues. It is important to note that these products are not categorized as medicines or drugs, and they have not received approval from the FDA for the prevention, treatment, or cure of any medical condition, ailment, or disease. We must emphasize that any form of bodily introduction of these products into humans or animals is strictly prohibited by law. It is essential to adhere to these guidelines to ensure compliance with legal and ethical standards in research and experimentation.