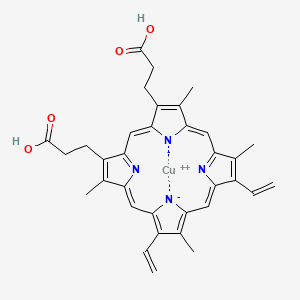
Cu(II) protoporphyrin IX
- Click on QUICK INQUIRY to receive a quote from our team of experts.
- With the quality product at a COMPETITIVE price, you can focus more on your research.
Overview
Description
Copper(II) protoporphyrin IX is a metalloporphyrin compound where a copper ion is coordinated to the protoporphyrin IX ring. Protoporphyrin IX is a naturally occurring porphyrin that plays a crucial role in biological systems, particularly as a precursor to heme, which is essential for oxygen transport in hemoglobin and myoglobin . Copper(II) protoporphyrin IX is known for its unique photophysical and electrochemical properties .
Preparation Methods
Synthetic Routes and Reaction Conditions
Copper(II) protoporphyrin IX can be synthesized through the reaction of protoporphyrin IX with copper salts. A common method involves dissolving protoporphyrin IX in a suitable solvent, such as dimethyl sulfoxide (DMSO), and then adding a copper(II) salt, such as copper(II) acetate. The reaction mixture is stirred at room temperature until the copper ion is fully coordinated to the protoporphyrin IX ring .
Industrial Production Methods
Industrial production of copper(II) protoporphyrin IX typically involves large-scale synthesis using similar methods as described above but optimized for higher yields and purity. The process may include additional purification steps, such as recrystallization or chromatography, to ensure the final product meets industrial standards .
Chemical Reactions Analysis
Types of Reactions
Copper(II) protoporphyrin IX undergoes various chemical reactions, including oxidation, reduction, and substitution reactions. These reactions are influenced by the presence of the copper ion and the conjugated system of the protoporphyrin IX ring .
Common Reagents and Conditions
Oxidation: Copper(II) protoporphyrin IX can be oxidized using oxidizing agents such as hydrogen peroxide or potassium permanganate.
Reduction: Reduction reactions can be carried out using reducing agents like sodium borohydride or hydrazine.
Major Products
The major products formed from these reactions depend on the specific reagents and conditions used. For example, oxidation may lead to the formation of copper(III) protoporphyrin IX, while reduction may yield copper(I) protoporphyrin IX .
Scientific Research Applications
Copper(II) protoporphyrin IX has a wide range of applications in scientific research:
Mechanism of Action
The mechanism of action of copper(II) protoporphyrin IX involves its ability to interact with molecular targets through coordination chemistry. The copper ion can participate in redox reactions, facilitating electron transfer processes. Additionally, the conjugated system of the protoporphyrin IX ring allows for efficient absorption and emission of light, making it useful in photodynamic therapy and optoelectronic applications .
Comparison with Similar Compounds
Similar Compounds
Zinc(II) protoporphyrin IX: Similar to copper(II) protoporphyrin IX but with a zinc ion instead of copper.
Iron(III) protoporphyrin IX (Heme): The iron-containing counterpart, essential for oxygen transport in hemoglobin and myoglobin.
Magnesium protoporphyrin IX: A precursor to chlorophyll, playing a crucial role in photosynthesis.
Uniqueness
Copper(II) protoporphyrin IX is unique due to its specific photophysical and electrochemical properties, which are not observed in its zinc or iron counterparts. The presence of the copper ion allows for unique interactions with light and electrons, making it particularly useful in applications such as photodynamic therapy and optoelectronics .
Biological Activity
Cu(II) protoporphyrin IX (Cu(II) PPIX) is a metalloporphyrin that has garnered attention in various biological and biochemical studies due to its unique properties and potential applications. This article explores its biological activity, mechanisms of action, and relevant case studies, providing a comprehensive overview based on diverse research findings.
Chemical Structure and Properties
This compound has the following chemical attributes:
- Molecular Formula : C₃₄H₃₂CuN₄O₄
- Molecular Weight : 624.188 g/mol
- CAS Number : 14494-37-2
The compound serves as a model for studying heme-like activities due to its structural resemblance to heme, which is crucial in many biological processes, including oxygen transport and electron transfer.
1. Role in Heme Oxygenase Inhibition
Cu(II) PPIX is known to act as a negative control in studies involving heme oxygenase (HO), an enzyme implicated in various physiological processes, including inflammation and vascular repair. Research indicates that Cu(II) PPIX can inhibit HO activity, which may contribute to tumor cell resistance against chemotherapy and modulate free radical formation .
2. Binding Properties
Studies utilizing resonance Raman spectroscopy have demonstrated that Cu(II) PPIX interacts with various proteins, including apomyoglobin. The binding characteristics reveal that Cu(II) is five-coordinate, likely coordinating with histidine residues within the protein structure. This interaction alters the heme environment and can affect the protein's functionality .
Case Study 1: Inhibition of Heme Oxygenase
A study investigated the effects of Cu(II) PPIX on HO activity in tumor cells. Results indicated that Cu(II) PPIX significantly inhibited HO, leading to reduced oxidative stress markers and enhanced sensitivity of cancer cells to chemotherapy agents. This suggests potential therapeutic applications for Cu(II) PPIX in cancer treatment .
Case Study 2: Metalloporphyrin Interactions with Ferrochelatase
Research on murine ferrochelatase revealed insights into how Cu(II) PPIX and other metal derivatives bind to the enzyme's active site. The findings showed that Cu(II) PPIX could serve as an inhibitor, affecting the enzyme's ability to chelate iron into protoporphyrin IX, which is essential for heme synthesis .
Comparative Analysis of Biological Activity
Compound | Biological Activity | Mechanism |
---|---|---|
This compound | Inhibits heme oxygenase activity | Interacts with histidine residues in proteins |
Zn(II) Protoporphyrin IX | Functions as a control for Cu(II) PPIX | Serves as an inhibitor for HO |
Fe(III) Protoporphyrin IX | Active in heme biosynthesis | Binds ferrous ions for heme formation |
Properties
Molecular Formula |
C34H32CuN4O4 |
---|---|
Molecular Weight |
624.2 g/mol |
IUPAC Name |
copper;3-[18-(2-carboxyethyl)-8,13-bis(ethenyl)-3,7,12,17-tetramethylporphyrin-21,23-diid-2-yl]propanoic acid |
InChI |
InChI=1S/C34H34N4O4.Cu/c1-7-21-17(3)25-13-26-19(5)23(9-11-33(39)40)31(37-26)16-32-24(10-12-34(41)42)20(6)28(38-32)15-30-22(8-2)18(4)27(36-30)14-29(21)35-25;/h7-8,13-16H,1-2,9-12H2,3-6H3,(H4,35,36,37,38,39,40,41,42);/q;+2/p-2 |
InChI Key |
ASFPSNQTLAUXFI-UHFFFAOYSA-L |
Canonical SMILES |
CC1=C(C2=CC3=NC(=CC4=C(C(=C([N-]4)C=C5C(=C(C(=N5)C=C1[N-]2)C)C=C)C)C=C)C(=C3CCC(=O)O)C)CCC(=O)O.[Cu+2] |
Origin of Product |
United States |
Disclaimer and Information on In-Vitro Research Products
Please be aware that all articles and product information presented on BenchChem are intended solely for informational purposes. The products available for purchase on BenchChem are specifically designed for in-vitro studies, which are conducted outside of living organisms. In-vitro studies, derived from the Latin term "in glass," involve experiments performed in controlled laboratory settings using cells or tissues. It is important to note that these products are not categorized as medicines or drugs, and they have not received approval from the FDA for the prevention, treatment, or cure of any medical condition, ailment, or disease. We must emphasize that any form of bodily introduction of these products into humans or animals is strictly prohibited by law. It is essential to adhere to these guidelines to ensure compliance with legal and ethical standards in research and experimentation.