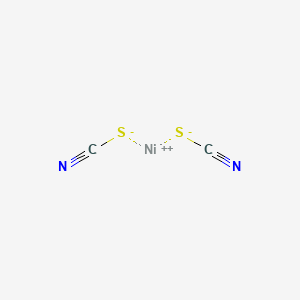
Nickel thiocyanate
Overview
Description
Nickel thiocyanate is a coordination polymer with the chemical formula Ni(SCN)₂. It is a green-brown solid that was first structurally characterized in 1982. The compound consists of two-dimensional sheets held together by Van der Waals forces, and it belongs to the mercury thiocyanate structure type. Each nickel ion is octahedrally coordinated by four sulfur atoms and two nitrogen atoms from the thiocyanate ligands .
Mechanism of Action
Target of Action
Nickel Thiocyanate primarily targets the nickel atom in its complexes . The nickel atom is octahedrally coordinated with two thiocyanate N atoms but with a different number of isonicotinamide N and water O atoms . This coordination is crucial for the compound’s function and interaction with its environment.
Mode of Action
This compound interacts with its targets through a process known as coordination . In all complexes, the nickel atom is octahedrally coordinated with two thiocyanate N atoms . The coordination can vary depending on the number of isonicotinamide N and water O atoms . This interaction results in changes in the compound’s structure and function.
Biochemical Pathways
This compound affects the biochemical pathways involved in the formation of its complexes. The compound’s interaction with its targets influences the formation of these complexes, which are crucial for its function
Pharmacokinetics
It’s known that the compound’s stoichiometry can be altered based on different ligand affinities towards nickel . The concentration of isonicotinamide strongly influences the number of coordinated isn ligands .
Result of Action
The result of this compound’s action is the formation of various complexes, which are characterized by different structures and functions . These complexes are formed as a compromise between the nucleation process, reactant ratios, ligand affinities, and intermolecular interactions in the crystal packing .
Action Environment
The action of this compound can be influenced by various environmental factors. For instance, the concentration of isonicotinamide in the environment can strongly influence the number of coordinated isn ligands . Additionally, the compound’s action can be affected by the presence of other substances in the environment, such as other ligands or co-crystallized moieties .
Biochemical Analysis
Biochemical Properties
Nickel thiocyanate plays a significant role in various biochemical reactions. It interacts with several enzymes and proteins, influencing their activity and function. One of the key interactions is with urease, an enzyme that catalyzes the hydrolysis of urea into ammonia and carbon dioxide. Nickel ions, including those from this compound, are essential cofactors for urease activity . Additionally, this compound can interact with other nickel-dependent enzymes such as [NiFe]-hydrogenase and carbon monoxide dehydrogenase, which are involved in energy metabolism and gas sensing . These interactions are primarily mediated through the binding of nickel ions to the active sites of these enzymes, facilitating their catalytic functions.
Cellular Effects
This compound has profound effects on various types of cells and cellular processes. It influences cell function by affecting cell signaling pathways, gene expression, and cellular metabolism. For instance, exposure to this compound can lead to changes in the expression of genes involved in metal ion transport and detoxification . This compound can also disrupt cellular redox balance, leading to oxidative stress and potential damage to cellular components . Furthermore, this compound has been shown to interfere with the normal functioning of mitochondria, affecting cellular energy production and metabolism .
Molecular Mechanism
The molecular mechanism of this compound involves its interaction with biomolecules at the molecular level. This compound can bind to proteins and enzymes, altering their structure and function. For example, the binding of nickel ions from this compound to the active sites of enzymes can either inhibit or activate their catalytic activity . This compound can also induce conformational changes in proteins, affecting their stability and interactions with other biomolecules . Additionally, this compound can influence gene expression by modulating the activity of transcription factors and other regulatory proteins .
Temporal Effects in Laboratory Settings
In laboratory settings, the effects of this compound can change over time due to its stability and degradation. Studies have shown that this compound is relatively stable under standard laboratory conditions, but it can degrade over time, especially under acidic or oxidative environments . Long-term exposure to this compound in in vitro or in vivo studies has revealed potential chronic effects on cellular function, including persistent oxidative stress and alterations in cellular metabolism . These temporal effects highlight the importance of considering the stability and degradation of this compound in experimental designs.
Dosage Effects in Animal Models
The effects of this compound vary with different dosages in animal models. At low doses, this compound can act as a micronutrient, supporting the activity of nickel-dependent enzymes and promoting normal cellular functions . At higher doses, this compound can become toxic, leading to adverse effects such as oxidative stress, inflammation, and tissue damage . Studies have identified threshold effects, where the toxicity of this compound becomes apparent only above certain concentration levels . These findings underscore the need for careful dosage considerations in experimental and therapeutic applications of this compound.
Metabolic Pathways
This compound is involved in several metabolic pathways, particularly those related to metal ion metabolism and detoxification. The compound can interact with enzymes such as thiocyanate hydrolase, which catalyzes the conversion of thiocyanate to carbonyl sulfide and cyanate . This interaction is crucial for the detoxification of thiocyanate and the maintenance of cellular redox balance . Additionally, this compound can influence metabolic fluxes by modulating the activity of key enzymes in energy metabolism and biosynthetic pathways .
Transport and Distribution
The transport and distribution of this compound within cells and tissues involve specific transporters and binding proteins. Nickel ions from this compound are transported across cell membranes by high-affinity nickel transporters, such as the nickel/cobalt transporter (NiCoT) family . These transporters facilitate the uptake and distribution of nickel ions to various cellular compartments, ensuring their availability for biochemical reactions . The localization and accumulation of this compound within cells are influenced by its interactions with binding proteins and other cellular components .
Subcellular Localization
This compound exhibits specific subcellular localization patterns that affect its activity and function. The compound is primarily localized in the vacuoles and cytoplasm of cells, where it forms complexes with organic acids and other ligands . This subcellular localization is essential for the detoxification and sequestration of nickel ions, preventing their toxic effects on cellular components . Additionally, this compound can be targeted to specific organelles through post-translational modifications and targeting signals, ensuring its proper distribution and function within cells .
Preparation Methods
Synthetic Routes and Reaction Conditions: Nickel thiocyanate can be synthesized via salt metathesis. One common method involves reacting methanolic solutions of potassium thiocyanate and nickel(II) perchlorate hexahydrate. The precipitated potassium perchlorate is filtered off, and the methanol is removed to yield pure microcrystalline this compound .
Another method involves treating a solution of nickel(II) chloride or nickel(II) nitrate in acetic acid with a solution of ammonium thiocyanate in the same solvent at room temperature. The product is then filtered, washed, and dried .
Industrial Production Methods: Industrial production methods for this compound are similar to laboratory synthesis, involving the reaction of nickel salts with thiocyanate salts under controlled conditions to ensure purity and yield.
Chemical Reactions Analysis
Types of Reactions: Nickel thiocyanate undergoes various chemical reactions, including:
Oxidation: this compound can be oxidized to form different nickel compounds.
Reduction: It can be reduced under specific conditions to yield nickel metal or other nickel-containing compounds.
Substitution: this compound can participate in substitution reactions where the thiocyanate ligands are replaced by other ligands.
Common Reagents and Conditions:
Oxidation: Common oxidizing agents include hydrogen peroxide and potassium permanganate.
Reduction: Reducing agents such as hydrogen gas or sodium borohydride can be used.
Substitution: Ligands like phosphines, amines, and other donor molecules can replace thiocyanate under appropriate conditions.
Major Products:
Oxidation: Nickel oxides or hydroxides.
Reduction: Nickel metal or nickel hydrides.
Substitution: Nickel complexes with new ligands, such as nickel phosphine complexes.
Scientific Research Applications
Catalytic Applications
Nickel thiocyanate serves as a catalyst in various chemical reactions due to its ability to form complexes with different ligands. It is particularly relevant in:
- Electrochemical Reactions : this compound has been used in electrochemical sensors and batteries due to its conductive properties and ability to facilitate electron transfer processes .
- Organic Synthesis : It acts as a catalyst in organic reactions such as the synthesis of isothiocyanates from amines and thiocyanates .
Material Science
In material science, this compound's coordination chemistry allows it to form interesting functional materials:
- Nanoparticle Synthesis : this compound nanoparticles have been developed for use in wound dressings, demonstrating antimicrobial properties and promoting rapid wound healing. Studies show that these nanoparticles are non-toxic up to certain concentrations and exhibit significant antibacterial activity .
- Complex Formation : Research has shown that this compound can form complexes with various ligands, influencing their structural and magnetic properties. For instance, complexes with isonicotinamide have been characterized using X-ray diffraction techniques, revealing unique hydrogen bonding patterns .
Biological Applications
This compound has garnered attention for its potential biological applications:
- Antimicrobial Properties : Recent studies indicate that this compound exhibits antimicrobial activity against various pathogens, making it a candidate for developing new antimicrobial agents .
- Toxicological Studies : Understanding the cytotoxicity of nickel compounds is crucial for assessing their safety in biomedical applications. Research indicates that while this compound can influence cellular processes, it requires careful evaluation to mitigate potential health risks associated with nickel exposure.
Environmental Chemistry
This compound's interactions with biological macromolecules have implications for environmental chemistry:
- Binding Studies : Investigations into how this compound binds with proteins and nucleic acids help elucidate its environmental impact and potential toxicity. Such studies are essential for understanding the compound's behavior in biological systems and ecosystems .
Comparative Analysis of this compound with Similar Compounds
The following table compares this compound with other transition metal thiocyanates based on unique features:
Compound | Formula | Unique Features |
---|---|---|
This compound | Ni(SCN)₂ | Exhibits distinct magnetic properties; forms various complexes |
Cobalt(II) Thiocyanate | Co(SCN)₂ | Different magnetic properties compared to nickel |
Copper(I) Thiocyanate | Cu(SCN) | Known for distinct structural characteristics |
Zinc Thiocyanate | Zn(SCN)₂ | Used in agriculture; different solubility compared to nickel |
Silver Thiocyanate | AgSCN | Highly soluble; used in photographic processes |
Case Study 1: Antimicrobial Wound Dressings
A study developed cotton gauze wound dressings impregnated with this compound nanoparticles. These dressings demonstrated rapid scarless healing in rat models while exhibiting antimicrobial properties against common pathogens. The study concluded that such dressings could provide a promising solution for treating various wounds effectively .
Case Study 2: Electrochemical Sensors
Research on electrochemical sensors utilizing this compound highlighted its effectiveness in detecting specific analytes. The sensors exhibited high sensitivity and selectivity due to the unique electronic properties of this compound when incorporated into the sensor matrix .
Comparison with Similar Compounds
Nickel thiocyanate can be compared with other nickel halides and thiocyanates:
Nickel(II) bromide, Nickel(II) chloride, Nickel(II) iodide: These compounds share similar coordination geometries but differ in their reactivity and applications.
Copper(I) thiocyanate, Cobalt(II) thiocyanate, Mercury(II) thiocyanate: These thiocyanates have different metal centers, leading to variations in their chemical properties and uses.
This compound is unique due to its specific coordination environment and its ability to form stable complexes with a variety of ligands, making it versatile for different applications.
Biological Activity
Nickel thiocyanate (Ni(SCN)₂) is a compound that has garnered attention in various fields of biological research due to its complex interactions with biological systems. This article provides a comprehensive overview of its biological activity, including toxicity, antimicrobial properties, and interactions with macromolecules.
This compound is a coordination compound formed from nickel and thiocyanate ions. It can be synthesized through various methods, including precipitation from nickel chloride solutions with potassium thiocyanate. The resulting compound often exhibits octahedral coordination geometry, where nickel is bonded to thiocyanate ligands and other coordinating molecules such as water or organic ligands .
1. Toxicity
Nickel compounds, including this compound, are known for their potential toxicity. Research indicates that exposure to nickel can lead to various adverse health effects:
- Cytotoxicity: this compound has been shown to exhibit cytotoxic effects on various cell lines, indicating its potential for inducing cellular damage.
- Respiratory Effects: Studies have highlighted that inhalation of nickel compounds can lead to respiratory inflammation and other lung-related issues. The National Toxicology Program (NTP) reported significant respiratory toxicity at specific concentrations .
- Carcinogenic Potential: Nickel compounds are classified as possible human carcinogens, with evidence suggesting that prolonged exposure may increase cancer risk .
Toxicity Parameter | Observed Effects | Reference |
---|---|---|
Cytotoxicity | Cell damage in vitro | |
Respiratory Effects | Lung inflammation | |
Carcinogenicity | Potential cancer risk |
2. Antimicrobial Properties
This compound has demonstrated antimicrobial activity against various pathogens. Its mechanisms of action are still under investigation but may involve:
- Disruption of Cellular Functions: Nickel ions can interfere with microbial cellular processes, leading to growth inhibition.
- Interaction with Macromolecules: The binding of this compound to proteins and nucleic acids may alter their functions, contributing to its antimicrobial effects .
Case Study Example:
A study conducted at the University of Mysore explored the antimicrobial efficacy of this compound against common bacterial strains. Results indicated significant inhibitory effects on both Gram-positive and Gram-negative bacteria, suggesting its potential application in antimicrobial therapies .
Interaction with Biological Macromolecules
This compound's interaction with proteins and nucleic acids poses both risks and potential therapeutic applications:
- Protein Binding: The compound can bind to proteins, potentially altering their structure and function. This interaction raises concerns regarding its safety in biomedical applications.
- Nucleic Acid Interaction: Studies suggest that this compound may interact with DNA, which could lead to mutagenic effects or influence gene expression.
Research Findings
Recent studies have focused on the synthesis of various nickel(II) thiocyanate complexes and their biological implications. For example:
- A series of complexes were synthesized using isonicotinamide as a co-ligand, revealing enhanced biological activity compared to simple this compound .
- Computational studies have provided insights into the stability and reactivity of these complexes, highlighting their potential use in drug development .
Properties
IUPAC Name |
nickel(2+);dithiocyanate | |
---|---|---|
Details | Computed by LexiChem 2.6.6 (PubChem release 2019.06.18) | |
Source | PubChem | |
URL | https://pubchem.ncbi.nlm.nih.gov | |
Description | Data deposited in or computed by PubChem | |
InChI |
InChI=1S/2CHNS.Ni/c2*2-1-3;/h2*3H;/q;;+2/p-2 | |
Details | Computed by InChI 1.0.5 (PubChem release 2019.06.18) | |
Source | PubChem | |
URL | https://pubchem.ncbi.nlm.nih.gov | |
Description | Data deposited in or computed by PubChem | |
InChI Key |
ALYMILAYQDOMFU-UHFFFAOYSA-L | |
Details | Computed by InChI 1.0.5 (PubChem release 2019.06.18) | |
Source | PubChem | |
URL | https://pubchem.ncbi.nlm.nih.gov | |
Description | Data deposited in or computed by PubChem | |
Canonical SMILES |
C(#N)[S-].C(#N)[S-].[Ni+2] | |
Details | Computed by OEChem 2.1.5 (PubChem release 2019.06.18) | |
Source | PubChem | |
URL | https://pubchem.ncbi.nlm.nih.gov | |
Description | Data deposited in or computed by PubChem | |
Molecular Formula |
C2N2NiS2 | |
Details | Computed by PubChem 2.1 (PubChem release 2019.06.18) | |
Source | PubChem | |
URL | https://pubchem.ncbi.nlm.nih.gov | |
Description | Data deposited in or computed by PubChem | |
DSSTOX Substance ID |
DTXSID30929573 | |
Record name | Nickel(2+) thiocyanate | |
Source | EPA DSSTox | |
URL | https://comptox.epa.gov/dashboard/DTXSID30929573 | |
Description | DSSTox provides a high quality public chemistry resource for supporting improved predictive toxicology. | |
Molecular Weight |
174.86 g/mol | |
Details | Computed by PubChem 2.1 (PubChem release 2021.05.07) | |
Source | PubChem | |
URL | https://pubchem.ncbi.nlm.nih.gov | |
Description | Data deposited in or computed by PubChem | |
CAS No. |
13689-92-4 | |
Record name | Nickel(2+) thiocyanate | |
Source | EPA DSSTox | |
URL | https://comptox.epa.gov/dashboard/DTXSID30929573 | |
Description | DSSTox provides a high quality public chemistry resource for supporting improved predictive toxicology. | |
Record name | Nickel dithiocyanate | |
Source | European Chemicals Agency (ECHA) | |
URL | https://echa.europa.eu/substance-information/-/substanceinfo/100.033.808 | |
Description | The European Chemicals Agency (ECHA) is an agency of the European Union which is the driving force among regulatory authorities in implementing the EU's groundbreaking chemicals legislation for the benefit of human health and the environment as well as for innovation and competitiveness. | |
Explanation | Use of the information, documents and data from the ECHA website is subject to the terms and conditions of this Legal Notice, and subject to other binding limitations provided for under applicable law, the information, documents and data made available on the ECHA website may be reproduced, distributed and/or used, totally or in part, for non-commercial purposes provided that ECHA is acknowledged as the source: "Source: European Chemicals Agency, http://echa.europa.eu/". Such acknowledgement must be included in each copy of the material. ECHA permits and encourages organisations and individuals to create links to the ECHA website under the following cumulative conditions: Links can only be made to webpages that provide a link to the Legal Notice page. | |
Retrosynthesis Analysis
AI-Powered Synthesis Planning: Our tool employs the Template_relevance Pistachio, Template_relevance Bkms_metabolic, Template_relevance Pistachio_ringbreaker, Template_relevance Reaxys, Template_relevance Reaxys_biocatalysis model, leveraging a vast database of chemical reactions to predict feasible synthetic routes.
One-Step Synthesis Focus: Specifically designed for one-step synthesis, it provides concise and direct routes for your target compounds, streamlining the synthesis process.
Accurate Predictions: Utilizing the extensive PISTACHIO, BKMS_METABOLIC, PISTACHIO_RINGBREAKER, REAXYS, REAXYS_BIOCATALYSIS database, our tool offers high-accuracy predictions, reflecting the latest in chemical research and data.
Strategy Settings
Precursor scoring | Relevance Heuristic |
---|---|
Min. plausibility | 0.01 |
Model | Template_relevance |
Template Set | Pistachio/Bkms_metabolic/Pistachio_ringbreaker/Reaxys/Reaxys_biocatalysis |
Top-N result to add to graph | 6 |
Feasible Synthetic Routes
Disclaimer and Information on In-Vitro Research Products
Please be aware that all articles and product information presented on BenchChem are intended solely for informational purposes. The products available for purchase on BenchChem are specifically designed for in-vitro studies, which are conducted outside of living organisms. In-vitro studies, derived from the Latin term "in glass," involve experiments performed in controlled laboratory settings using cells or tissues. It is important to note that these products are not categorized as medicines or drugs, and they have not received approval from the FDA for the prevention, treatment, or cure of any medical condition, ailment, or disease. We must emphasize that any form of bodily introduction of these products into humans or animals is strictly prohibited by law. It is essential to adhere to these guidelines to ensure compliance with legal and ethical standards in research and experimentation.