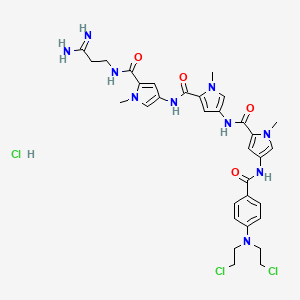
Tallimustine hydrochloride
- Click on QUICK INQUIRY to receive a quote from our team of experts.
- With the quality product at a COMPETITIVE price, you can focus more on your research.
Overview
Description
SB 272844 is a selective antagonist of the C-X-C chemokine receptor type 2 (CXCR2). This compound has been studied for its potential therapeutic applications, particularly in the context of inflammatory diseases and cancer. By blocking the CXCR2 receptor, SB 272844 can inhibit the action of certain chemokines, which are signaling proteins involved in the recruitment of immune cells to sites of inflammation or tumor growth .
Preparation Methods
Synthetic Routes and Reaction Conditions
The synthesis of SB 272844 involves several key steps, including the formation of the core structure and the introduction of specific functional groups. The exact synthetic route and reaction conditions are proprietary and not publicly disclosed in detail. general synthetic methods for similar compounds typically involve:
Formation of the Core Structure: This step often involves the use of cyclization reactions to form the core ring structure of the compound.
Functional Group Introduction: Specific functional groups are introduced through various chemical reactions, such as alkylation, acylation, or halogenation.
Purification: The final product is purified using techniques such as chromatography to ensure the desired purity and yield.
Industrial Production Methods
Industrial production of SB 272844 would likely involve scaling up the laboratory synthesis methods to larger reactors and optimizing the reaction conditions for higher yields and purity. This process would also include rigorous quality control measures to ensure consistency and safety of the final product.
Chemical Reactions Analysis
Types of Reactions
SB 272844 can undergo various chemical reactions, including:
Oxidation: The compound can be oxidized under specific conditions to form oxidized derivatives.
Reduction: Reduction reactions can be used to modify certain functional groups within the molecule.
Substitution: Substitution reactions, such as nucleophilic or electrophilic substitution, can be employed to replace specific atoms or groups within the compound.
Common Reagents and Conditions
Common reagents used in these reactions include oxidizing agents (e.g., hydrogen peroxide), reducing agents (e.g., sodium borohydride), and various nucleophiles or electrophiles for substitution reactions. The reaction conditions, such as temperature, solvent, and pH, are carefully controlled to achieve the desired transformations.
Major Products
The major products formed from these reactions depend on the specific reagents and conditions used. For example, oxidation of SB 272844 may yield oxidized derivatives with altered biological activity, while substitution reactions can introduce new functional groups that modify the compound’s properties.
Scientific Research Applications
Chemistry: In chemical research, SB 272844 serves as a valuable tool for studying the role of CXCR2 in various biological processes.
Biology: The compound is used to investigate the mechanisms of chemokine signaling and its impact on immune cell recruitment and function.
Industry: In the pharmaceutical industry, SB 272844 is explored as a lead compound for developing new anti-inflammatory and anticancer drugs.
Mechanism of Action
SB 272844 exerts its effects by selectively binding to the CXCR2 receptor, thereby blocking the interaction between the receptor and its ligands, such as interleukin-8 and growth-related oncogene-alpha . This inhibition prevents the activation of downstream signaling pathways that lead to the recruitment and activation of neutrophils and other immune cells. By blocking these pathways, SB 272844 can reduce inflammation and potentially inhibit tumor growth.
Comparison with Similar Compounds
Similar Compounds
Several other compounds are known to target the CXCR2 receptor, including:
SB 225002: Another selective CXCR2 antagonist with similar biological activity.
Reparixin: A non-competitive allosteric inhibitor of CXCR2.
Danirixin: A selective CXCR2 antagonist being investigated for its potential in treating respiratory diseases.
Uniqueness of SB 272844
SB 272844 is unique in its high selectivity for the CXCR2 receptor and its potent inhibitory effects on chemokine-mediated neutrophil recruitment. This selectivity makes it a valuable tool for studying the specific role of CXCR2 in various biological processes and for developing targeted therapies with minimal off-target effects.
Properties
CAS No. |
118438-45-2 |
---|---|
Molecular Formula |
C32H39Cl3N10O4 |
Molecular Weight |
734.1 g/mol |
IUPAC Name |
N-[5-[[5-[(3-amino-3-iminopropyl)carbamoyl]-1-methylpyrrol-3-yl]carbamoyl]-1-methylpyrrol-3-yl]-4-[[4-[bis(2-chloroethyl)amino]benzoyl]amino]-1-methylpyrrole-2-carboxamide;hydrochloride |
InChI |
InChI=1S/C32H38Cl2N10O4.ClH/c1-41-18-22(14-25(41)30(46)37-11-8-28(35)36)39-32(48)27-16-23(19-43(27)3)40-31(47)26-15-21(17-42(26)2)38-29(45)20-4-6-24(7-5-20)44(12-9-33)13-10-34;/h4-7,14-19H,8-13H2,1-3H3,(H3,35,36)(H,37,46)(H,38,45)(H,39,48)(H,40,47);1H |
InChI Key |
BLSOATWWAGIRGE-UHFFFAOYSA-N |
SMILES |
CN1C=C(C=C1C(=O)NC2=CN(C(=C2)C(=O)NC3=CN(C(=C3)C(=O)NCCC(=N)N)C)C)NC(=O)C4=CC=C(C=C4)N(CCCl)CCCl.Cl |
Canonical SMILES |
CN1C=C(C=C1C(=O)NC2=CN(C(=C2)C(=O)NC3=CN(C(=C3)C(=O)NCCC(=N)N)C)C)NC(=O)C4=CC=C(C=C4)N(CCCl)CCCl.Cl |
Synonyms |
FCE 24517 FCE-24517 N-deformyl-N-(4-N-N,N-bis(2-choroethylamino)benzoyl)distamycin tallimustine |
Origin of Product |
United States |
Disclaimer and Information on In-Vitro Research Products
Please be aware that all articles and product information presented on BenchChem are intended solely for informational purposes. The products available for purchase on BenchChem are specifically designed for in-vitro studies, which are conducted outside of living organisms. In-vitro studies, derived from the Latin term "in glass," involve experiments performed in controlled laboratory settings using cells or tissues. It is important to note that these products are not categorized as medicines or drugs, and they have not received approval from the FDA for the prevention, treatment, or cure of any medical condition, ailment, or disease. We must emphasize that any form of bodily introduction of these products into humans or animals is strictly prohibited by law. It is essential to adhere to these guidelines to ensure compliance with legal and ethical standards in research and experimentation.