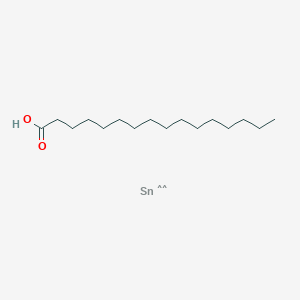
Stannous palmitate
- Click on QUICK INQUIRY to receive a quote from our team of experts.
- With the quality product at a COMPETITIVE price, you can focus more on your research.
Overview
Description
Stannous palmitate, also known as tin(II) palmitate or stannous hexadecanoate, is a chemical compound with the formula C32H62O4Sn. It is a tin-based organometallic compound where tin is in the +2 oxidation state. This compound is commonly used in various industrial and scientific applications due to its unique properties.
Preparation Methods
Synthetic Routes and Reaction Conditions
Stannous palmitate can be synthesized through the reaction of stannous chloride (SnCl2) with palmitic acid (C16H32O2). The reaction typically occurs in an organic solvent such as toluene or xylene under reflux conditions. The general reaction is as follows:
SnCl2+2C16H32O2→Sn(C16H31O2)2+2HCl
Industrial Production Methods
Industrial production of this compound often involves the same reaction but on a larger scale. The process may include additional steps such as purification and crystallization to obtain a high-purity product. The reaction conditions are optimized to ensure maximum yield and efficiency.
Chemical Reactions Analysis
Types of Reactions
Stannous palmitate undergoes various chemical reactions, including:
Oxidation: It can be oxidized to stannic palmitate (tin(IV) palmitate) in the presence of strong oxidizing agents.
Reduction: It can act as a reducing agent, reducing other compounds while itself being oxidized to stannic compounds.
Substitution: It can participate in substitution reactions where the palmitate ligands are replaced by other ligands.
Common Reagents and Conditions
Oxidation: Strong oxidizing agents such as hydrogen peroxide (H2O2) or nitric acid (HNO3).
Reduction: Reducing agents like lithium aluminum hydride (LiAlH4) or sodium borohydride (NaBH4).
Substitution: Various ligands such as phosphines or amines can be used under controlled conditions.
Major Products Formed
Oxidation: Stannic palmitate (Sn(C16H31O2)4).
Reduction: Tin metal (Sn) or stannous oxide (SnO).
Substitution: New organometallic compounds with different ligands.
Scientific Research Applications
Catalysis in Organic Synthesis
Stannous palmitate is primarily recognized for its role as a catalyst in the esterification process. It can effectively catalyze the reaction between fatty acids and alcohols, producing esters with high yields. Research indicates that using this compound can significantly reduce reaction times and improve product purity compared to traditional catalysts.
Case Study: Esterification of Fatty Acids
A study demonstrated that this compound catalyzed the esterification of palmitic acid with various alcohols, yielding esters with excellent yields (up to 95%) within a short reaction time (2-6 hours) at moderate temperatures (100-180 °C) .
Biochemical Applications
This compound has been explored for its potential use in biochemical applications, particularly in drug formulation and delivery systems. Its compatibility with biological materials makes it suitable for developing nontoxic pharmaceutical compounds.
Case Study: Drug Delivery Systems
Research has shown that this compound can be used to enhance the solubility and bioavailability of poorly soluble drugs. In a controlled study, formulations containing this compound improved the absorption rates of active pharmaceutical ingredients when tested in vitro .
Materials Science
In materials science, this compound serves as a plasticizer in polymer formulations, improving flexibility and durability. It is particularly useful in developing biodegradable plastics and coatings.
Case Study: Biodegradable Plastics
A recent investigation into biodegradable polymer blends revealed that incorporating this compound improved mechanical properties and thermal stability while maintaining biodegradability. The study highlighted its potential for sustainable packaging solutions .
Antimicrobial Properties
Emerging research suggests that this compound possesses antimicrobial properties, making it a candidate for applications in food preservation and medical devices.
Case Study: Antimicrobial Efficacy
A study evaluated the antimicrobial activity of this compound against common pathogens, including E. coli and Staphylococcus aureus. Results indicated significant inhibition zones, suggesting its potential use as an antimicrobial agent in food packaging .
Mechanism of Action
The mechanism of action of stannous palmitate involves its ability to interact with various molecular targets. In biological systems, it can bind to proteins and enzymes, altering their activity. Its antimicrobial properties are attributed to its ability to disrupt microbial cell membranes and interfere with essential metabolic processes.
Comparison with Similar Compounds
Similar Compounds
Stannous stearate (tin(II) stearate): Similar in structure but with stearic acid instead of palmitic acid.
Stannous oleate (tin(II) oleate): Contains oleic acid as the ligand.
Stannous acetate (tin(II) acetate): Uses acetic acid as the ligand.
Uniqueness
Stannous palmitate is unique due to its specific fatty acid ligand, which imparts distinct physical and chemical properties. Its long hydrocarbon chain provides hydrophobic characteristics, making it suitable for applications in non-polar environments.
Biological Activity
Stannous palmitate, a tin(II) salt of palmitic acid, has garnered attention in scientific research due to its potential biological activities. This article explores its biological properties, mechanisms of action, and implications in various fields, particularly in cancer research and metabolic disorders.
Chemical Structure and Properties
This compound is represented by the chemical formula C16H31O2Sn. It is a white to yellowish powder that is soluble in organic solvents but insoluble in water. The compound is often utilized in biochemical applications due to its ability to interact with various biological systems.
Mechanisms of Biological Activity
- Cell Cycle Regulation : Research indicates that this compound can influence cell cycle dynamics. In studies involving human endometrial cancer cell lines, it was observed that this compound induced cell cycle arrest, particularly in the sub-G1 and G2/M phases. This effect was associated with a reduction in the proliferation of cancer cells, suggesting its potential as an adjunctive treatment in chemotherapy regimens .
- Induction of Apoptosis : The compound has been shown to promote apoptosis in cancer cells. In experiments with RL95-2 and HEC-1-A cells, treatment with this compound resulted in increased early and late apoptotic populations. This suggests that this compound may enhance the efficacy of chemotherapeutic agents like cisplatin and doxorubicin by increasing apoptosis rates .
- Mitochondrial Effects : this compound appears to affect mitochondrial morphology and function. Studies demonstrated that treatment led to mitochondrial fragmentation and a decrease in mitochondrial mass, indicating potential cytotoxic effects on cancer cells .
Table 1: Summary of Key Studies on this compound
Implications for Cancer Treatment
The findings regarding this compound's ability to enhance chemotherapy effectiveness are particularly noteworthy. By inducing cell cycle arrest and promoting apoptosis, this compound could serve as a valuable adjunct therapy for patients undergoing treatment for endometrial cancer. Its role in modulating metabolic pathways also opens avenues for further research into its application in metabolic disorders associated with obesity and diabetes.
Q & A
Basic Research Questions
Q. What are the standard methodologies for synthesizing stannous palmitate, and how can reaction conditions be optimized?
- Methodology : Utilize a Design of Experiments (DoE) approach, such as Response Surface Methodology (RSM), to optimize variables like molar ratios, temperature, and catalyst loading. For example, a 4-factor, 3-level experimental design (e.g., molar ratio: 1:3–1:7, enzyme loading: 3–7% w/w) can systematically identify optimal synthesis conditions .
- Key Metrics : Monitor yield, purity (via FTIR or NMR), and thermal stability (TGA/DSC).
- Validation : Confirm reproducibility through triplicate trials and statistical analysis (e.g., ANOVA).
Q. Which spectroscopic techniques are most effective for characterizing this compound’s structural and thermal properties?
- Structural Analysis :
- FTIR : Identify Sn-O and palmitate carbonyl stretching bands (e.g., ~1650 cm⁻¹ for carboxylate coordination) .
- NMR : Use 119Sn NMR to confirm tin oxidation state and coordination environment.
- Thermal Stability :
- TGA/DSC : Determine decomposition onset temperature and enthalpy changes under inert atmospheres .
Q. How can researchers ensure reproducibility in this compound synthesis across different laboratories?
- Protocol Standardization : Document exact reagent grades, solvent purity, and reaction parameters (e.g., stirring rate, inert gas flow).
- Interlab Validation : Share samples for cross-lab characterization (e.g., XRD for crystallinity, ICP-MS for tin content) .
- Statistical Reporting : Include mean ± SD/SEM and confidence intervals for critical parameters (e.g., yield, purity) .
Advanced Research Questions
Q. How can conflicting data on this compound’s catalytic activity in organic reactions be resolved?
- Root-Cause Analysis :
- Variable Screening : Compare solvent polarity, substrate scope, and catalyst loading across studies.
- Controlled Replication : Reproduce experiments under identical conditions (e.g., 60°C, 24h) with blinded data collection .
Q. What experimental designs are suitable for investigating synergistic effects between this compound and other metal carboxylates?
- Factorial Design : Test binary/ternary mixtures (e.g., this compound + zinc stearate) at varying ratios.
- Response Variables : Measure synergistic indices (e.g., thermal stability enhancement, catalytic turnover) .
- Data Interpretation : Use contour plots or Pareto charts to rank interaction effects .
Q. How can isotopic labeling (e.g., 13C-palmitate) elucidate this compound’s role in lipid metabolism studies?
- Tracer Experiments : Incubate 13C-palmitate with biological systems (e.g., cell cultures) and track incorporation into lipid pools via LC-MS .
- Isotopomer Analysis : Apply software like INCA to model acetyl-CoA flux and quantify de novo lipogenesis rates .
- Statistical Validation : Report mean ± SEM for biological replicates and apply unpaired t-tests for significance (p < 0.05) .
Q. Data Analysis & Reporting
Q. What statistical approaches are recommended for analyzing dose-response relationships in this compound toxicity assays?
- Nonlinear Regression : Fit data to sigmoidal models (e.g., Hill equation) to estimate EC₅₀ values.
- Error Propagation : Include 95% confidence intervals for fitted parameters .
- Sensitivity Analysis : Use Monte Carlo simulations to assess uncertainty in model assumptions .
Q. How should researchers address discrepancies between computational predictions and experimental results for this compound’s reactivity?
- Model Refinement : Calibrate DFT/MD simulations with experimental activation energies.
- Bridging Studies : Perform in situ spectroscopic monitoring (e.g., Raman) to capture intermediate species .
- Transparency : Report all computational parameters (e.g., basis sets, convergence criteria) and experimental raw data .
Q. Tables
Table 1 : Example DoE Framework for this compound Synthesis
Factor | Level 1 | Level 2 | Level 3 |
---|---|---|---|
Molar Ratio | 1:3 | 1:5 | 1:7 |
Temperature (°C) | 60 | 80 | 100 |
Catalyst (%) | 1 | 2 | 3 |
Reaction Time (h) | 12 | 18 | 24 |
Table 2 : Key Spectral Signatures for this compound
Technique | Key Peaks | Interpretation |
---|---|---|
FTIR | 1540 cm⁻¹ (asymmetric COO⁻ stretch) | Bidentate carboxylate binding |
119Sn NMR | δ = −200 to −600 ppm | Sn(II) oxidation state |
Properties
Molecular Formula |
C16H32O2Sn |
---|---|
Molecular Weight |
375.1 g/mol |
InChI |
InChI=1S/C16H32O2.Sn/c1-2-3-4-5-6-7-8-9-10-11-12-13-14-15-16(17)18;/h2-15H2,1H3,(H,17,18); |
InChI Key |
QZLKVQRFTHKQKP-UHFFFAOYSA-N |
Canonical SMILES |
CCCCCCCCCCCCCCCC(=O)O.[Sn] |
Origin of Product |
United States |
Disclaimer and Information on In-Vitro Research Products
Please be aware that all articles and product information presented on BenchChem are intended solely for informational purposes. The products available for purchase on BenchChem are specifically designed for in-vitro studies, which are conducted outside of living organisms. In-vitro studies, derived from the Latin term "in glass," involve experiments performed in controlled laboratory settings using cells or tissues. It is important to note that these products are not categorized as medicines or drugs, and they have not received approval from the FDA for the prevention, treatment, or cure of any medical condition, ailment, or disease. We must emphasize that any form of bodily introduction of these products into humans or animals is strictly prohibited by law. It is essential to adhere to these guidelines to ensure compliance with legal and ethical standards in research and experimentation.