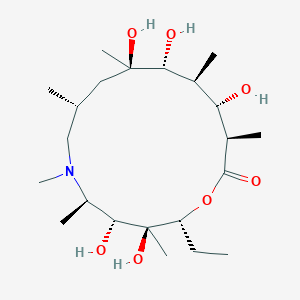
Azithromycin aglycone
- Click on QUICK INQUIRY to receive a quote from our team of experts.
- With the quality product at a COMPETITIVE price, you can focus more on your research.
Overview
Description
Azithromycin aglycone is a derivative of azithromycin, a well-known macrolide antibiotic. Azithromycin is part of the azalide subclass of macrolides, characterized by a 15-membered ring structure. The aglycone form refers to the molecule without its sugar moiety, which can significantly alter its chemical properties and biological activities .
Preparation Methods
Synthetic Routes and Reaction Conditions: Azithromycin aglycone can be synthesized from erythromycin A through a series of chemical reactions. One common method involves treating erythromycin A with hydroxylamine hydrochloride and a base in methanol at reflux temperature for 10 hours to form an oxime . This is followed by further chemical modifications to obtain the aglycone form.
Industrial Production Methods: Industrial production of this compound typically involves large-scale synthesis using similar chemical routes but optimized for efficiency and yield. The process may include steps like solvent evaporation and crystallization to purify the final product .
Chemical Reactions Analysis
Types of Reactions: Azithromycin aglycone undergoes various chemical reactions, including:
Oxidation: This reaction can modify the aglycone structure, potentially altering its biological activity.
Reduction: Reduction reactions can be used to modify specific functional groups within the molecule.
Substitution: Common in the synthesis of derivatives, substitution reactions involve replacing one functional group with another.
Common Reagents and Conditions:
Oxidation: Reagents like potassium permanganate or hydrogen peroxide under acidic or basic conditions.
Reduction: Reagents such as sodium borohydride or lithium aluminum hydride.
Substitution: Various halogenating agents or nucleophiles under controlled conditions.
Major Products: The major products formed from these reactions depend on the specific reagents and conditions used. For example, oxidation might yield hydroxylated derivatives, while substitution could produce a variety of functionalized this compound molecules .
Scientific Research Applications
Azithromycin aglycone has a wide range of applications in scientific research:
Chemistry: Used as a starting material for the synthesis of new macrolide derivatives with potential therapeutic applications.
Biology: Studied for its interactions with bacterial ribosomes and its role in inhibiting protein synthesis.
Medicine: Investigated for its antibacterial properties and potential use in treating infections resistant to other antibiotics.
Industry: Utilized in the development of novel drug delivery systems to enhance the bioavailability and efficacy of azithromycin
Mechanism of Action
Azithromycin aglycone exerts its effects by binding to the 23S rRNA of the bacterial 50S ribosomal subunit. This binding inhibits the transpeptidation and translocation steps of protein synthesis, effectively stopping bacterial growth. The aglycone form may have different binding affinities and specificities compared to the parent compound, azithromycin .
Comparison with Similar Compounds
Erythromycin: A 14-membered macrolide antibiotic from which azithromycin is derived.
Clarithromycin: Another macrolide with a similar structure but different pharmacokinetic properties.
Telithromycin: A ketolide antibiotic with a modified macrolide structure for improved activity against resistant strains.
Uniqueness: Azithromycin aglycone is unique due to its 15-membered ring structure and the absence of sugar moieties, which can influence its solubility, stability, and biological activity. Compared to erythromycin and clarithromycin, azithromycin and its aglycone form have improved acid stability and a broader spectrum of activity .
Biological Activity
Azithromycin, a member of the azalide class of antibiotics, is derived from erythromycin and exhibits unique biological activities due to its aglycone structure. This article delves into the biological activity of azithromycin aglycone, focusing on its mechanisms of action, pharmacokinetics, antimicrobial efficacy, immunomodulatory effects, and resistance patterns.
Azithromycin's structure features an aza-methyl substitution in the 15-membered aglycone ring, which enhances its stability and broad-spectrum antibacterial activity compared to other macrolides. The primary mechanism of action involves the inhibition of bacterial protein synthesis by binding to the 23S rRNA of the 50S ribosomal subunit, disrupting the transpeptidation and translocation steps essential for protein synthesis . This leads to bacteriostatic effects against a wide array of pathogens.
Table 1: Mechanisms of Action of Azithromycin
Mechanism | Description |
---|---|
Protein Synthesis Inhibition | Binds to ribosomal subunits, blocking protein synthesis. |
Quorum Sensing Inhibition | Disrupts bacterial communication systems. |
Biofilm Formation Reduction | Inhibits biofilm development in various bacterial species. |
Immunomodulation | Modulates immune responses and reduces inflammation. |
Pharmacokinetics
Azithromycin exhibits unique pharmacokinetic properties characterized by rapid tissue penetration and prolonged retention in tissues. Following administration, it achieves high concentrations in lung tissues, which are significantly higher than serum levels, making it effective for respiratory infections . The area under the curve (AUC) for lung tissue increases proportionally with dosage, indicating predictable pharmacokinetics that enhance therapeutic efficacy.
Table 2: Pharmacokinetic Parameters of Azithromycin
Parameter | Value (500 mg dose) | Value (1000 mg dose) |
---|---|---|
Cmax (mg/kg) | 8.93 ± 2.05 | 18.6 ± 2.20 |
AUC (h × mg/kg) | 1245.4 | 2514.2 |
Tissue Concentration | >2 mg/kg | >4 mg/kg |
Antimicrobial Activity
Azithromycin demonstrates a broad spectrum of activity against both Gram-positive and Gram-negative bacteria, including significant intracellular pathogens such as Chlamydia trachomatis and Legionella pneumophila. It is particularly effective against Haemophilus influenzae, which is resistant to many older macrolides .
Case Study: Efficacy Against Respiratory Infections
A study evaluating azithromycin's effectiveness in treating respiratory tract infections found that it significantly reduced symptoms and bacterial load in patients with pneumonia caused by susceptible strains of Streptococcus pneumoniae. The study highlighted that higher tissue concentrations correlated with better clinical outcomes .
Immunomodulatory Effects
Beyond its antibacterial properties, azithromycin exhibits immunomodulatory effects that are beneficial in chronic inflammatory conditions such as asthma and cystic fibrosis. It reduces pro-inflammatory cytokine production and alters macrophage polarization, contributing to improved lung function and reduced exacerbations in chronic respiratory diseases .
Resistance Patterns
Despite its efficacy, the emergence of resistance to azithromycin is a growing concern. Studies indicate an increasing prevalence of resistant strains due to inappropriate use and over-prescribing . Monitoring resistance patterns is crucial for maintaining the drug's clinical utility.
Table 3: Resistance Patterns Observed
Pathogen | Resistance Rate (%) |
---|---|
Streptococcus pneumoniae | 30-40 |
Haemophilus influenzae | 15-25 |
Chlamydia trachomatis | <5 |
Properties
CAS No. |
117693-42-2 |
---|---|
Molecular Formula |
C22H43NO7 |
Molecular Weight |
433.6 g/mol |
IUPAC Name |
(2R,3S,4R,5R,8R,10R,11R,12S,13S,14R)-2-ethyl-3,4,10,11,13-pentahydroxy-3,5,6,8,10,12,14-heptamethyl-1-oxa-6-azacyclopentadecan-15-one |
InChI |
InChI=1S/C22H43NO7/c1-9-16-22(7,29)19(26)15(5)23(8)11-12(2)10-21(6,28)18(25)13(3)17(24)14(4)20(27)30-16/h12-19,24-26,28-29H,9-11H2,1-8H3/t12-,13+,14-,15-,16-,17+,18-,19-,21-,22-/m1/s1 |
InChI Key |
DZMMYAUQTDKEJG-DFKIMAAQSA-N |
Isomeric SMILES |
CC[C@@H]1[C@@]([C@@H]([C@H](N(C[C@@H](C[C@@]([C@@H]([C@H]([C@@H]([C@H](C(=O)O1)C)O)C)O)(C)O)C)C)C)O)(C)O |
Canonical SMILES |
CCC1C(C(C(N(CC(CC(C(C(C(C(C(=O)O1)C)O)C)O)(C)O)C)C)C)O)(C)O |
Origin of Product |
United States |
Disclaimer and Information on In-Vitro Research Products
Please be aware that all articles and product information presented on BenchChem are intended solely for informational purposes. The products available for purchase on BenchChem are specifically designed for in-vitro studies, which are conducted outside of living organisms. In-vitro studies, derived from the Latin term "in glass," involve experiments performed in controlled laboratory settings using cells or tissues. It is important to note that these products are not categorized as medicines or drugs, and they have not received approval from the FDA for the prevention, treatment, or cure of any medical condition, ailment, or disease. We must emphasize that any form of bodily introduction of these products into humans or animals is strictly prohibited by law. It is essential to adhere to these guidelines to ensure compliance with legal and ethical standards in research and experimentation.