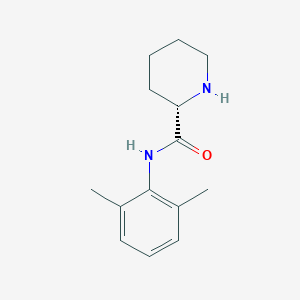
(S)-N-(2,6-Dimethylphenyl)-2-piperidinecarboxamide
Overview
Description
(S)-N-(2,6-Dimethylphenyl)-2-piperidinecarboxamide is a chemical compound known for its significant applications in various scientific fields. This compound is characterized by its specific stereochemistry, which plays a crucial role in its biological and chemical properties.
Preparation Methods
Synthetic Routes and Reaction Conditions: The synthesis of (S)-N-(2,6-Dimethylphenyl)-2-piperidinecarboxamide typically involves the following steps:
Starting Materials: The synthesis begins with 2,6-dimethylphenylamine and (S)-2-piperidinecarboxylic acid.
Coupling Reaction: The primary step involves the coupling of 2,6-dimethylphenylamine with (S)-2-piperidinecarboxylic acid using a coupling reagent such as dicyclohexylcarbodiimide (DCC) in the presence of a catalyst like 4-dimethylaminopyridine (DMAP).
Purification: The resulting product is purified through recrystallization or chromatography to obtain the desired this compound.
Industrial Production Methods: In industrial settings, the production of this compound may involve large-scale batch reactors with optimized reaction conditions to ensure high yield and purity. The use of automated systems for monitoring and controlling reaction parameters is common to maintain consistency and efficiency.
Types of Reactions:
Oxidation: this compound can undergo oxidation reactions, typically using oxidizing agents like potassium permanganate or chromium trioxide.
Reduction: Reduction reactions can be carried out using reducing agents such as lithium aluminum hydride or sodium borohydride.
Substitution: The compound can participate in substitution reactions, where functional groups are replaced by other groups under specific conditions.
Common Reagents and Conditions:
Oxidation: Potassium permanganate in acidic or neutral medium.
Reduction: Lithium aluminum hydride in anhydrous ether.
Substitution: Halogenating agents like thionyl chloride for chlorination.
Major Products:
Oxidation: Formation of corresponding carboxylic acids or ketones.
Reduction: Formation of amines or alcohols.
Substitution: Formation of halogenated derivatives.
Scientific Research Applications
(S)-N-(2,6-Dimethylphenyl)-2-piperidinecarboxamide has diverse applications in scientific research:
Chemistry: Used as a building block in organic synthesis for the preparation of more complex molecules.
Biology: Studied for its potential biological activities, including enzyme inhibition and receptor binding.
Medicine: Investigated for its pharmacological properties, particularly in the development of analgesic and anesthetic agents.
Industry: Utilized in the production of specialty chemicals and intermediates for pharmaceuticals.
Mechanism of Action
The mechanism of action of (S)-N-(2,6-Dimethylphenyl)-2-piperidinecarboxamide involves its interaction with specific molecular targets, such as enzymes or receptors. The compound’s stereochemistry allows it to fit into the active sites of these targets, modulating their activity. This interaction can lead to inhibition or activation of biochemical pathways, resulting in the compound’s observed effects.
Comparison with Similar Compounds
®-N-(2,6-Dimethylphenyl)-2-piperidinecarboxamide: The enantiomer of the compound with different stereochemistry.
N-(2,6-Dimethylphenyl)-2-piperidinecarboxamide: The racemic mixture containing both (S) and ® enantiomers.
Uniqueness: (S)-N-(2,6-Dimethylphenyl)-2-piperidinecarboxamide is unique due to its specific stereochemistry, which imparts distinct biological and chemical properties compared to its enantiomer and racemic mixture. This uniqueness is crucial in applications where stereospecific interactions are essential, such as in drug development and biochemical research.
Biological Activity
(S)-N-(2,6-Dimethylphenyl)-2-piperidinecarboxamide, commonly known as ropivacaine, is a local anesthetic that has garnered attention for its pharmacological properties, particularly in the fields of anesthesiology and neurology. This article explores its biological activity, structure-activity relationships (SAR), and potential therapeutic applications.
Chemical Structure and Properties
Ropivacaine is a chiral compound characterized by the presence of a piperidine ring and a dimethylphenyl group. Its molecular formula is with a molecular weight of approximately 246.35 g/mol. The compound exists primarily in its (S)-enantiomeric form, which has been shown to exhibit superior anesthetic properties compared to its racemic counterpart.
Ropivacaine functions by blocking sodium channels in neuronal cell membranes, leading to a decrease in the propagation of action potentials. This mechanism is critical for its effectiveness as a local anesthetic. The compound's selectivity for sensory nerves over motor nerves contributes to its favorable safety profile, reducing the risk of motor block during surgical procedures.
Anesthetic Properties
Ropivacaine demonstrates significant analgesic effects with a lower cardiotoxic risk compared to other local anesthetics like bupivacaine. Studies indicate that ropivacaine provides effective pain relief in various surgical contexts, including orthopedic and obstetric procedures .
Anticonvulsant Activity
Research has also explored the anticonvulsant potential of ropivacaine and its analogs. In animal models, compounds related to ropivacaine have shown efficacy in the maximal electroshock seizure (MES) test, indicating potential use in epilepsy management. For instance, modifications in the structure of piperidine derivatives have led to increased anticonvulsant activity without enhancing neurotoxicity .
Structure-Activity Relationships (SAR)
The biological activity of ropivacaine can be influenced by various structural modifications:
- Substituents on the Aromatic Ring : Introduction of halogen groups (e.g., Cl) on the aromatic ring has been associated with enhanced anticonvulsant activity.
- Piperidine Ring Modifications : Alterations to the piperidine structure affect both efficacy and toxicity. For example, replacing the piperidine ring with cycloalkane structures generally results in decreased activity and increased neurotoxicity .
- Amide Group Positioning : Moving the carboxamide group within the piperidine framework can significantly alter the compound's potency and safety profile .
Case Studies
- Clinical Application in Surgery : Ropivacaine has been successfully employed in various surgical settings. A study evaluating its use in tonsillectomy procedures reported significant reductions in postoperative pain scores when compared to placebo treatments .
- Anticonvulsant Research : In a study focusing on piperidine derivatives, ropivacaine analogs exhibited promising results in reducing seizure frequency in animal models, suggesting their potential role as anticonvulsants .
Comparative Efficacy Table
Compound | ED50 (mg/kg) | TD50 (mg/kg) | PI (Protective Index) |
---|---|---|---|
Ropivacaine | 5.8 | 36.4 | 6.3 |
Bupivacaine | 4.0 | 25.0 | 6.25 |
Other Piperidine Derivatives | Varies | Varies | Varies |
ED50 = Effective Dose for 50% response; TD50 = Toxic Dose for 50% response; PI = Protective Index.
Properties
IUPAC Name |
(2S)-N-(2,6-dimethylphenyl)piperidine-2-carboxamide | |
---|---|---|
Source | PubChem | |
URL | https://pubchem.ncbi.nlm.nih.gov | |
Description | Data deposited in or computed by PubChem | |
InChI |
InChI=1S/C14H20N2O/c1-10-6-5-7-11(2)13(10)16-14(17)12-8-3-4-9-15-12/h5-7,12,15H,3-4,8-9H2,1-2H3,(H,16,17)/t12-/m0/s1 | |
Source | PubChem | |
URL | https://pubchem.ncbi.nlm.nih.gov | |
Description | Data deposited in or computed by PubChem | |
InChI Key |
SILRCGDPZGQJOQ-LBPRGKRZSA-N | |
Source | PubChem | |
URL | https://pubchem.ncbi.nlm.nih.gov | |
Description | Data deposited in or computed by PubChem | |
Canonical SMILES |
CC1=C(C(=CC=C1)C)NC(=O)C2CCCCN2 | |
Source | PubChem | |
URL | https://pubchem.ncbi.nlm.nih.gov | |
Description | Data deposited in or computed by PubChem | |
Isomeric SMILES |
CC1=C(C(=CC=C1)C)NC(=O)[C@@H]2CCCCN2 | |
Source | PubChem | |
URL | https://pubchem.ncbi.nlm.nih.gov | |
Description | Data deposited in or computed by PubChem | |
Molecular Formula |
C14H20N2O | |
Source | PubChem | |
URL | https://pubchem.ncbi.nlm.nih.gov | |
Description | Data deposited in or computed by PubChem | |
DSSTOX Substance ID |
DTXSID10181713 | |
Record name | 2',6'-Pipecoloxylidide, (+)- | |
Source | EPA DSSTox | |
URL | https://comptox.epa.gov/dashboard/DTXSID10181713 | |
Description | DSSTox provides a high quality public chemistry resource for supporting improved predictive toxicology. | |
Molecular Weight |
232.32 g/mol | |
Source | PubChem | |
URL | https://pubchem.ncbi.nlm.nih.gov | |
Description | Data deposited in or computed by PubChem | |
CAS No. |
27262-40-4 | |
Record name | 2′,6′-Pipecoloxylidide, (+)- | |
Source | CAS Common Chemistry | |
URL | https://commonchemistry.cas.org/detail?cas_rn=27262-40-4 | |
Description | CAS Common Chemistry is an open community resource for accessing chemical information. Nearly 500,000 chemical substances from CAS REGISTRY cover areas of community interest, including common and frequently regulated chemicals, and those relevant to high school and undergraduate chemistry classes. This chemical information, curated by our expert scientists, is provided in alignment with our mission as a division of the American Chemical Society. | |
Explanation | The data from CAS Common Chemistry is provided under a CC-BY-NC 4.0 license, unless otherwise stated. | |
Record name | 2',6'-Pipecoloxylidide, (+)- | |
Source | ChemIDplus | |
URL | https://pubchem.ncbi.nlm.nih.gov/substance/?source=chemidplus&sourceid=0027262404 | |
Description | ChemIDplus is a free, web search system that provides access to the structure and nomenclature authority files used for the identification of chemical substances cited in National Library of Medicine (NLM) databases, including the TOXNET system. | |
Record name | 2',6'-Pipecoloxylidide, (+)- | |
Source | EPA DSSTox | |
URL | https://comptox.epa.gov/dashboard/DTXSID10181713 | |
Description | DSSTox provides a high quality public chemistry resource for supporting improved predictive toxicology. | |
Record name | 2-Piperidinecarboxamide, N-(2,6-dimethylphenyl)-, (2S) | |
Source | European Chemicals Agency (ECHA) | |
URL | https://echa.europa.eu/information-on-chemicals | |
Description | The European Chemicals Agency (ECHA) is an agency of the European Union which is the driving force among regulatory authorities in implementing the EU's groundbreaking chemicals legislation for the benefit of human health and the environment as well as for innovation and competitiveness. | |
Explanation | Use of the information, documents and data from the ECHA website is subject to the terms and conditions of this Legal Notice, and subject to other binding limitations provided for under applicable law, the information, documents and data made available on the ECHA website may be reproduced, distributed and/or used, totally or in part, for non-commercial purposes provided that ECHA is acknowledged as the source: "Source: European Chemicals Agency, http://echa.europa.eu/". Such acknowledgement must be included in each copy of the material. ECHA permits and encourages organisations and individuals to create links to the ECHA website under the following cumulative conditions: Links can only be made to webpages that provide a link to the Legal Notice page. | |
Record name | 2',6'-PIPECOLOXYLIDIDE, (+)- | |
Source | FDA Global Substance Registration System (GSRS) | |
URL | https://gsrs.ncats.nih.gov/ginas/app/beta/substances/4A8HC6M4AL | |
Description | The FDA Global Substance Registration System (GSRS) enables the efficient and accurate exchange of information on what substances are in regulated products. Instead of relying on names, which vary across regulatory domains, countries, and regions, the GSRS knowledge base makes it possible for substances to be defined by standardized, scientific descriptions. | |
Explanation | Unless otherwise noted, the contents of the FDA website (www.fda.gov), both text and graphics, are not copyrighted. They are in the public domain and may be republished, reprinted and otherwise used freely by anyone without the need to obtain permission from FDA. Credit to the U.S. Food and Drug Administration as the source is appreciated but not required. | |
Retrosynthesis Analysis
AI-Powered Synthesis Planning: Our tool employs the Template_relevance Pistachio, Template_relevance Bkms_metabolic, Template_relevance Pistachio_ringbreaker, Template_relevance Reaxys, Template_relevance Reaxys_biocatalysis model, leveraging a vast database of chemical reactions to predict feasible synthetic routes.
One-Step Synthesis Focus: Specifically designed for one-step synthesis, it provides concise and direct routes for your target compounds, streamlining the synthesis process.
Accurate Predictions: Utilizing the extensive PISTACHIO, BKMS_METABOLIC, PISTACHIO_RINGBREAKER, REAXYS, REAXYS_BIOCATALYSIS database, our tool offers high-accuracy predictions, reflecting the latest in chemical research and data.
Strategy Settings
Precursor scoring | Relevance Heuristic |
---|---|
Min. plausibility | 0.01 |
Model | Template_relevance |
Template Set | Pistachio/Bkms_metabolic/Pistachio_ringbreaker/Reaxys/Reaxys_biocatalysis |
Top-N result to add to graph | 6 |
Feasible Synthetic Routes
Q1: What is the significance of (S)-N-(2,6-Dimethylphenyl)-2-piperidinecarboxamide in pharmaceutical synthesis?
A: this compound serves as a crucial chiral intermediate in the production of levobupivacaine hydrochloride []. This compound is significant because it possesses the desired (S)-stereochemistry, which is essential for the pharmacological activity of levobupivacaine as a local anesthetic.
Q2: How does the synthesis process impact the quality and yield of levobupivacaine hydrochloride?
A: The synthesis process detailed in the research [] highlights the importance of optimizing each step to ensure high purity and yield of levobupivacaine hydrochloride. Specifically, the use of (R,S)-N-(2,6-dimethylphenyl)piperidine-2-carboxamide as the starting material and l-(–)-dibenzoyl tartaric acid for chiral separation allows for the isolation of the desired (S)-enantiomer with high enantiomeric excess (ee) values of 99.30%. This efficient chiral resolution directly contributes to the final product's high chemical purity (99.90%) and overall yield (45% over three steps). The researchers also emphasize the safety and scalability of their method, which are crucial factors for industrial production.
Disclaimer and Information on In-Vitro Research Products
Please be aware that all articles and product information presented on BenchChem are intended solely for informational purposes. The products available for purchase on BenchChem are specifically designed for in-vitro studies, which are conducted outside of living organisms. In-vitro studies, derived from the Latin term "in glass," involve experiments performed in controlled laboratory settings using cells or tissues. It is important to note that these products are not categorized as medicines or drugs, and they have not received approval from the FDA for the prevention, treatment, or cure of any medical condition, ailment, or disease. We must emphasize that any form of bodily introduction of these products into humans or animals is strictly prohibited by law. It is essential to adhere to these guidelines to ensure compliance with legal and ethical standards in research and experimentation.