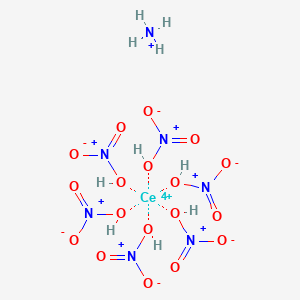
Azanium;cerium(4+);nitric acid
Overview
Description
Azanium;cerium(4+);nitric acid is a compound that consists of azanium (a protonated amine), cerium in its +4 oxidation state, and nitric acid Cerium is a rare earth metal known for its catalytic properties and is often used in various industrial applications Nitric acid is a strong acid commonly used in the production of fertilizers, explosives, and other chemicals
Mechanism of Action
Target of Action
Azanium;cerium(4+);nitric acid, also known as Ceric Ammonium Nitrate (CAN), is a specialized oxidizing agent used in organic synthesis . The primary targets of CAN are various functional groups in organic compounds, including alcohols, phenols, ethers, and C-H bonds, especially those that are benzylic .
Mode of Action
CAN acts as a strong one-electron oxidizing agent . In terms of its redox potential, it is an even stronger oxidizing agent than Cl2 . During the redox process, Ce(IV) in CAN is converted to Ce(III), a one-electron change, signaled by the fading of the solution color from orange to a pale yellow .
Biochemical Pathways
For example, alkenes undergo dinitroxylation, quinones are produced from catechols and hydroquinones, and even nitroalkanes are oxidized . These reactions can lead to significant changes in the structure and properties of the targeted compounds.
Pharmacokinetics
It is known that can is a water-soluble compound , which suggests that it could be readily absorbed and distributed in aqueous environments. The exact ADME (Absorption, Distribution, Metabolism, and Excretion) properties of CAN would depend on the specific conditions of its use.
Result of Action
The result of CAN’s action is the oxidation of targeted functional groups in organic compounds. This can lead to significant changes in the structure and properties of these compounds. For example, the oxidation of alkenes can result in the formation of dinitroxy compounds . The exact molecular and cellular effects of CAN’s action would depend on the specific compounds being targeted.
Biochemical Analysis
Biochemical Properties
Azanium;cerium(4+);nitric acid is known to interact with various biomolecules. For instance, cerium oxide nanoparticles have been shown to interact with reactive oxygen species (ROS), playing a role in modulating oxidative stress . This interaction with ROS can influence various biochemical reactions within the cell. Furthermore, cerium oxide nanoparticles have been reported to exhibit antioxidant as well as prooxidant activities .
Cellular Effects
The effects of this compound on cells are multifaceted. Cerium oxide nanoparticles have been shown to protect cells by reducing oxidative stress due to their redox activity . This can influence cell function, including impacts on cell signaling pathways, gene expression, and cellular metabolism. For instance, cerium oxide nanoparticles can facilitate the pro-oxidant properties of mesoporous silica nanoparticles, leading to increased ROS levels and the killing of cancer cells .
Molecular Mechanism
The molecular mechanism of action of this compound involves its strong oxidizing properties. In terms of its redox potential, it is an even stronger oxidizing agent than chlorine . In the redox process, Ce (IV) is converted to Ce (III), a one-electron change, signaled by the fading of the solution color from orange to a pale yellow .
Temporal Effects in Laboratory Settings
It is known that cerium oxide nanoparticles prepared from cerium ammonium nitrate have a significantly smaller particle size and higher conductivity . This suggests that the compound may have long-term effects on cellular function, potentially influencing the stability and degradation of the compound in in vitro or in vivo studies.
Dosage Effects in Animal Models
It is known that nanoparticles, including those of cerium oxide, can have varying effects depending on the dosage
Metabolic Pathways
Cerium oxide nanoparticles have been shown to interact with various components of cellular metabolism, including radicals, enzymes, proteins, transcription factors, and metabolites .
Transport and Distribution
Cerium oxide nanoparticles have been shown to have a smaller particle size and higher conductivity when prepared from cerium ammonium nitrate , suggesting that they may be readily transported and distributed within cells.
Preparation Methods
Synthetic Routes and Reaction Conditions
Azanium;cerium(4+);nitric acid can be synthesized by dissolving cerium oxide (CeO2) in hot and concentrated nitric acid. The reaction typically involves the following steps:
- Dissolution of cerium oxide in concentrated nitric acid.
- Formation of the hexanitratocerate(IV) anion [Ce(NO3)6]2−.
- Addition of ammonium ions to form the final compound.
The reaction conditions include maintaining a high temperature and using concentrated nitric acid to ensure complete dissolution of cerium oxide and formation of the desired product .
Industrial Production Methods
In industrial settings, the production of this compound involves large-scale dissolution of cerium oxide in nitric acid, followed by crystallization to obtain the pure compound. The process is carefully controlled to ensure high yield and purity of the final product .
Chemical Reactions Analysis
Types of Reactions
Azanium;cerium(4+);nitric acid undergoes various types of chemical reactions, including:
Oxidation: Cerium(4+) is a strong oxidizing agent and can oxidize various organic and inorganic compounds.
Reduction: Cerium(4+) can be reduced to cerium(3+) in the presence of suitable reducing agents.
Substitution: The nitrate groups in the compound can be substituted with other ligands under appropriate conditions.
Common Reagents and Conditions
Oxidation Reactions: Common reagents include alcohols, phenols, and ethers.
Reduction Reactions: Reducing agents such as oxalic acid can be used to reduce cerium(4+) to cerium(3+).
Substitution Reactions: Ligands such as sulfate or phosphate can replace nitrate groups under specific conditions.
Major Products Formed
Oxidation: Products include aldehydes, ketones, quinones, and nitroalkanes.
Reduction: The major product is cerium(3+) compounds.
Substitution: Products include cerium sulfate and cerium phosphate.
Scientific Research Applications
Azanium;cerium(4+);nitric acid has numerous applications in scientific research, including:
Comparison with Similar Compounds
Similar Compounds
Cerium(III) nitrate: Contains cerium in the +3 oxidation state and is used in similar applications but has different redox properties.
Cerium(IV) oxide: Another cerium(4+) compound used in catalysis and as an oxygen storage material.
Cerium(IV) sulfate: Formed by substituting nitrate groups with sulfate, used in various chemical reactions.
Uniqueness
Azanium;cerium(4+);nitric acid is unique due to its strong oxidizing properties and the ability to participate in a wide range of chemical reactions. Its combination of cerium(4+) and nitric acid makes it a versatile compound in both research and industrial applications .
Properties
IUPAC Name |
azanium;cerium(4+);nitric acid | |
---|---|---|
Source | PubChem | |
URL | https://pubchem.ncbi.nlm.nih.gov | |
Description | Data deposited in or computed by PubChem | |
InChI |
InChI=1S/Ce.6HNO3.H3N/c;6*2-1(3)4;/h;6*(H,2,3,4);1H3/q+4;;;;;;;/p+1 | |
Source | PubChem | |
URL | https://pubchem.ncbi.nlm.nih.gov | |
Description | Data deposited in or computed by PubChem | |
InChI Key |
LEXAATUBLOUJCN-UHFFFAOYSA-O | |
Source | PubChem | |
URL | https://pubchem.ncbi.nlm.nih.gov | |
Description | Data deposited in or computed by PubChem | |
Canonical SMILES |
[NH4+].[N+](=O)(O)[O-].[N+](=O)(O)[O-].[N+](=O)(O)[O-].[N+](=O)(O)[O-].[N+](=O)(O)[O-].[N+](=O)(O)[O-].[Ce+4] | |
Source | PubChem | |
URL | https://pubchem.ncbi.nlm.nih.gov | |
Description | Data deposited in or computed by PubChem | |
Molecular Formula |
CeH10N7O18+5 | |
Source | PubChem | |
URL | https://pubchem.ncbi.nlm.nih.gov | |
Description | Data deposited in or computed by PubChem | |
Molecular Weight |
536.23 g/mol | |
Source | PubChem | |
URL | https://pubchem.ncbi.nlm.nih.gov | |
Description | Data deposited in or computed by PubChem | |
CAS No. |
16774-21-3 | |
Record name | Ammonium ceric nitrate | |
Source | DTP/NCI | |
URL | https://dtp.cancer.gov/dtpstandard/servlet/dwindex?searchtype=NSC&outputformat=html&searchlist=4313 | |
Description | The NCI Development Therapeutics Program (DTP) provides services and resources to the academic and private-sector research communities worldwide to facilitate the discovery and development of new cancer therapeutic agents. | |
Explanation | Unless otherwise indicated, all text within NCI products is free of copyright and may be reused without our permission. Credit the National Cancer Institute as the source. | |
Retrosynthesis Analysis
AI-Powered Synthesis Planning: Our tool employs the Template_relevance Pistachio, Template_relevance Bkms_metabolic, Template_relevance Pistachio_ringbreaker, Template_relevance Reaxys, Template_relevance Reaxys_biocatalysis model, leveraging a vast database of chemical reactions to predict feasible synthetic routes.
One-Step Synthesis Focus: Specifically designed for one-step synthesis, it provides concise and direct routes for your target compounds, streamlining the synthesis process.
Accurate Predictions: Utilizing the extensive PISTACHIO, BKMS_METABOLIC, PISTACHIO_RINGBREAKER, REAXYS, REAXYS_BIOCATALYSIS database, our tool offers high-accuracy predictions, reflecting the latest in chemical research and data.
Strategy Settings
Precursor scoring | Relevance Heuristic |
---|---|
Min. plausibility | 0.01 |
Model | Template_relevance |
Template Set | Pistachio/Bkms_metabolic/Pistachio_ringbreaker/Reaxys/Reaxys_biocatalysis |
Top-N result to add to graph | 6 |
Feasible Synthetic Routes
Disclaimer and Information on In-Vitro Research Products
Please be aware that all articles and product information presented on BenchChem are intended solely for informational purposes. The products available for purchase on BenchChem are specifically designed for in-vitro studies, which are conducted outside of living organisms. In-vitro studies, derived from the Latin term "in glass," involve experiments performed in controlled laboratory settings using cells or tissues. It is important to note that these products are not categorized as medicines or drugs, and they have not received approval from the FDA for the prevention, treatment, or cure of any medical condition, ailment, or disease. We must emphasize that any form of bodily introduction of these products into humans or animals is strictly prohibited by law. It is essential to adhere to these guidelines to ensure compliance with legal and ethical standards in research and experimentation.