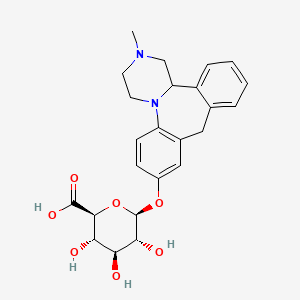
8-Hydroxymianserin glucuronide
- Click on QUICK INQUIRY to receive a quote from our team of experts.
- With the quality product at a COMPETITIVE price, you can focus more on your research.
Overview
Description
Beta-D-Glucopyranosiduronic acid, 1,2,3,4,10,14b-hexahydro-2-methyldibenzo[c,f]pyrazino[1,2-a]azepin-8-yl: is a complex organic compound that belongs to the class of glucopyranosiduronic acids. This compound is characterized by its unique structure, which includes a glucopyranosiduronic acid moiety linked to a hexahydro-dibenzo-pyrazino-azepin scaffold. The compound is of significant interest in various fields of scientific research due to its potential biological and chemical properties.
Preparation Methods
Synthetic Routes and Reaction Conditions: The synthesis of beta-D-Glucopyranosiduronic acid, 1,2,3,4,10,14b-hexahydro-2-methyldibenzo[c,f]pyrazino[1,2-a]azepin-8-yl involves multiple steps, including the protection and deprotection of functional groups, glycosylation, and cyclization reactions. One common approach involves the regioselective benzoylation of unprotected beta-glycopyranosides with benzoyl cyanide and an amine catalyst . This method allows for the selective protection of hydroxyl groups, facilitating subsequent synthetic steps.
Industrial Production Methods: Industrial production of this compound may involve optimized synthetic routes that ensure high yield and purity. These methods typically include large-scale glycosylation reactions, followed by purification steps such as crystallization or chromatography to isolate the desired product.
Chemical Reactions Analysis
Types of Reactions: Beta-D-Glucopyranosiduronic acid, 1,2,3,4,10,14b-hexahydro-2-methyldibenzo[c,f]pyrazino[1,2-a]azepin-8-yl undergoes various chemical reactions, including:
Oxidation: The compound can be oxidized to form corresponding carboxylic acids or ketones.
Reduction: Reduction reactions can convert carbonyl groups to alcohols.
Substitution: Nucleophilic substitution reactions can introduce different functional groups into the molecule.
Common Reagents and Conditions:
Oxidation: Common oxidizing agents include potassium permanganate (KMnO4) and chromium trioxide (CrO3).
Reduction: Reducing agents such as sodium borohydride (NaBH4) and lithium aluminum hydride (LiAlH4) are frequently used.
Substitution: Reagents like alkyl halides and nucleophiles (e.g., amines, thiols) are used under basic or acidic conditions.
Major Products: The major products formed from these reactions depend on the specific conditions and reagents used. For example, oxidation may yield carboxylic acids, while reduction can produce alcohols.
Scientific Research Applications
Pharmacological Applications
1. Understanding Metabolism and Pharmacokinetics
8-Hydroxymianserin glucuronide is crucial for studying the metabolic pathways of mianserin. The glucuronidation process enhances the solubility and excretion of the parent compound, which is essential for understanding its pharmacokinetic properties. Research has shown that glucuronides often play a role in drug metabolism, affecting drug efficacy and safety profiles .
2. Therapeutic Potential
Research indicates that this compound may contribute to the therapeutic effects of mianserin in treating depression and insomnia. By elucidating the interactions of this metabolite with neurotransmitter systems, researchers can better understand its potential benefits and side effects .
Toxicological Applications
1. Biomarker for Drug Monitoring
Due to its presence as a metabolite in biological fluids, this compound can serve as a biomarker for therapeutic drug monitoring. This application is vital in ensuring optimal dosing and minimizing adverse effects in patients undergoing treatment with mianserin .
2. Safety Assessment
The compound's role in toxicity studies is significant, particularly in assessing the safety of mianserin and similar drugs. Understanding how this metabolite behaves in biological systems can help predict potential toxicological outcomes .
Analytical Chemistry Applications
1. Method Development
this compound is utilized in analytical method development for detecting and quantifying drug metabolites in biological samples. Techniques such as liquid chromatography coupled with mass spectrometry (LC-MS) are commonly employed to achieve high sensitivity and specificity .
2. Quality Control
In pharmaceutical manufacturing, this compound is involved in quality control processes to ensure that products meet regulatory standards. Its analysis helps verify the consistency and purity of drug formulations containing mianserin .
Case Studies
Several case studies have investigated the implications of this compound in clinical settings:
-
Case Study 1: Therapeutic Drug Monitoring
A clinical study monitored patients treated with mianserin, measuring levels of this compound to adjust dosages effectively based on metabolic responses. -
Case Study 2: Toxicity Assessment
Research focused on patients experiencing adverse reactions while on mianserin therapy highlighted the importance of monitoring this compound levels to understand individual variability in drug metabolism.
Data Table: Summary of Key Findings
Mechanism of Action
The mechanism of action of beta-D-Glucopyranosiduronic acid, 1,2,3,4,10,14b-hexahydro-2-methyldibenzo[c,f]pyrazino[1,2-a]azepin-8-yl involves its interaction with specific molecular targets and pathways. The compound may inhibit enzymes involved in inflammatory processes, such as cyclooxygenase (COX) and nitric oxide synthase (NOS), thereby reducing the production of pro-inflammatory mediators . Additionally, it may interfere with cellular signaling pathways, leading to the modulation of gene expression and cellular responses.
Comparison with Similar Compounds
Phenyl beta-D-glucopyranoside: Known for its anti-inflammatory and anti-cancer activities.
Beta-D-glucopyranosiduronic acid, methyl, methyl ester, 2,3,4-triacetate: Used in carbohydrate chemistry and glycobiology research.
P-Nitrophenyl-beta-D-glucuronide: Utilized as a substrate for enzyme assays.
Uniqueness: Beta-D-Glucopyranosiduronic acid, 1,2,3,4,10,14b-hexahydro-2-methyldibenzo[c,f]pyrazino[1,2-a]azepin-8-yl stands out due to its complex structure, which combines a glucopyranosiduronic acid moiety with a hexahydro-dibenzo-pyrazino-azepin scaffold. This unique combination imparts distinct chemical and biological properties, making it a valuable compound for various research applications.
Biological Activity
8-Hydroxymianserin glucuronide is a metabolite derived from the pharmacological agent mirtazapine, which is primarily used as an antidepressant. This compound has garnered attention due to its potential biological activities, particularly in the context of cancer treatment and antimicrobial properties. This article delves into the biological activity of this compound, exploring its mechanisms, effects, and relevant research findings.
Chemical Structure and Properties
This compound is characterized by its glucuronidation, a process that enhances the solubility and excretion of compounds in biological systems. The molecular formula is C17H23N4O9P, with a molecular weight of approximately 458.1269 g/mol. Its structure allows for interaction with various biological targets, which is crucial for its activity.
The biological activity of this compound primarily involves its interaction with neurotransmitter systems and potential anticancer pathways:
- Antidepressant Activity : As a metabolite of mirtazapine, it may retain some properties that modulate serotonin and norepinephrine levels in the brain.
- Anticancer Properties : Research indicates that this compound may exhibit cytotoxic effects against various cancer cell lines, including breast cancer (MCF-7) and choriocarcinoma (Jeg-3) cells. The compound has been shown to significantly inhibit cell proliferation, suggesting a mechanism that may involve apoptosis or cell cycle arrest .
In Vitro Studies
Several studies have investigated the cytotoxic effects of this compound:
- Cytotoxicity Assays : In vitro assays using the MTT method have demonstrated that this compound exhibits significant cytotoxicity towards cancer cell lines. For instance, one study reported an IC50 value of approximately 26.02 μg/ml for MCF-7 cells .
- Mechanistic Insights : The compound's mechanism appears to involve the modulation of signaling pathways related to cell survival and apoptosis. For example, it has been noted to inhibit Jeg-3 cell growth by up to 62% compared to untreated controls .
Antimicrobial Activity
In addition to its anticancer properties, this compound has been studied for its antimicrobial effects:
- Antibacterial Activity : Preliminary findings suggest that it may possess antibacterial properties against certain pathogens, although specific data on the spectrum of activity remains limited.
Case Studies and Clinical Relevance
Case studies involving mirtazapine and its metabolites highlight the relevance of this compound in clinical settings:
- Biotransformation Studies : Research on the biotransformation of mirtazapine has shown that this compound is formed through metabolic processes in the liver, indicating its potential systemic effects following administration .
- Pharmacological Implications : Understanding the pharmacokinetics and dynamics of this metabolite can inform therapeutic strategies, especially in patients receiving mirtazapine for depression who may also have comorbid conditions like cancer.
Comparative Analysis with Similar Compounds
Compound Name | Molecular Formula | IC50 (μg/ml) | Primary Activity |
---|---|---|---|
This compound | C17H23N4O9P | 26.02 | Anticancer |
Mirtazapine | C17H19N3 | N/A | Antidepressant |
Norviburtinal | C16H20N2O3 | N/A | Anticancer |
Properties
Molecular Formula |
C24H28N2O7 |
---|---|
Molecular Weight |
456.5 g/mol |
IUPAC Name |
(2S,3S,4S,5R,6S)-3,4,5-trihydroxy-6-[(5-methyl-2,5-diazatetracyclo[13.4.0.02,7.08,13]nonadeca-1(15),8,10,12,16,18-hexaen-17-yl)oxy]oxane-2-carboxylic acid |
InChI |
InChI=1S/C24H28N2O7/c1-25-8-9-26-17-7-6-15(32-24-21(29)19(27)20(28)22(33-24)23(30)31)11-14(17)10-13-4-2-3-5-16(13)18(26)12-25/h2-7,11,18-22,24,27-29H,8-10,12H2,1H3,(H,30,31)/t18?,19-,20-,21+,22-,24+/m0/s1 |
InChI Key |
BIPZSOCAFBEZTQ-JZOWGYKDSA-N |
Isomeric SMILES |
CN1CCN2C(C1)C3=CC=CC=C3CC4=C2C=CC(=C4)O[C@H]5[C@@H]([C@H]([C@@H]([C@H](O5)C(=O)O)O)O)O |
Canonical SMILES |
CN1CCN2C(C1)C3=CC=CC=C3CC4=C2C=CC(=C4)OC5C(C(C(C(O5)C(=O)O)O)O)O |
Origin of Product |
United States |
Disclaimer and Information on In-Vitro Research Products
Please be aware that all articles and product information presented on BenchChem are intended solely for informational purposes. The products available for purchase on BenchChem are specifically designed for in-vitro studies, which are conducted outside of living organisms. In-vitro studies, derived from the Latin term "in glass," involve experiments performed in controlled laboratory settings using cells or tissues. It is important to note that these products are not categorized as medicines or drugs, and they have not received approval from the FDA for the prevention, treatment, or cure of any medical condition, ailment, or disease. We must emphasize that any form of bodily introduction of these products into humans or animals is strictly prohibited by law. It is essential to adhere to these guidelines to ensure compliance with legal and ethical standards in research and experimentation.