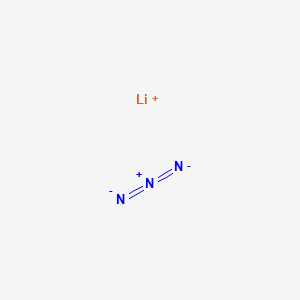
Lithium azide
Overview
Description
Lithium azide (LiN₃) is an inorganic compound composed of lithium (Li⁺) and the azide anion (N₃⁻). It has a molecular weight of 48.96 g/mol and a monoclinic crystal structure under standard conditions . The compound is highly reactive and decomposes upon heating to produce lithium and nitrogen gas, a characteristic shared with other metal azides . This compound is synthesized via metathesis reactions, such as the interaction of sodium azide (NaN₃) with lithium sulfate (Li₂SO₄) or lithium nitrate (LiNO₃) in aqueous solutions . Its applications span organic synthesis, where it serves as a precursor for triazole derivatives in electrocatalysis , and materials science, though its instability limits widespread industrial use compared to other azides.
Preparation Methods
Lithium azide can be synthesized through several methods:
Metathesis Reaction: This involves the reaction between sodium azide and lithium nitrate or lithium sulfate solutions
Reaction with Barium Azide: Lithium sulfate reacts with barium azide to produce this compound and barium sulfate
These reactions are typically carried out in aqueous solutions under controlled conditions to ensure the purity and yield of this compound .
Chemical Reactions Analysis
Thermal Decomposition
Lithium azide decomposes exothermically when heated, producing lithium metal and nitrogen gas. The reaction is temperature-dependent and exhibits distinct kinetic phases:
Key Findings:
-
Decomposition Temperature :
-
Kinetics :
Condition | Product | Temperature Range | Activation Energy (kJ/mol) |
---|---|---|---|
Rapid heating | Li + N₂ | 115–298°C | 13.1–17.6 |
Slow heating | Li + N₂ | 298°C | 41.4–48.1 |
Photolytic Decomposition
Exposure to ultraviolet (UV) light induces decomposition via a free-radical mechanism:
Key Findings:
-
Mechanism :
-
Environmental Sensitivity :
Substitution Reactions
The azide ion () acts as a nucleophile in reactions with electrophiles:
Example Reactions:
-
Alkylation :
-
Aryl Functionalization :
Reduction Reactions
This compound undergoes reduction to form lithium amide () and nitrogen gas:
Methods:
-
From Sodium Azide :
-
From Barium Azide :
Reactivity with Acids
This compound reacts vigorously with acids to release hydrazoic acid ():
Scientific Research Applications
Electrolyte Additive in Lithium-Sulfur Batteries
Lithium azide has been identified as a promising electrolyte additive for all-solid-state lithium-sulfur (Li-S) batteries. Its incorporation significantly enhances the cycling performance and energy efficiency of these batteries. The mechanism involves the formation of a compact, conductive passivation layer on the lithium metal anode, which mitigates issues such as dendrite formation and polysulfide shuttling. Studies indicate that this compound outperforms traditional additives like lithium nitrate (LiNO₃) in terms of stability and efficiency .
Key Findings:
- Enhanced Performance: this compound improves cycling performance and Coulombic efficiency.
- Dendrite Prevention: It forms a protective layer on the lithium anode, preventing dendrite growth.
- Comparative Advantage: Outperforms state-of-the-art additives in solid-state lithium-sulfur batteries.
High-Pressure Research
Research into the structural and electronic properties of this compound under high pressure has revealed significant insights into its behavior as a material. Studies using density-functional theory have shown that this compound exhibits anisotropic compressibility, with the b-axis being more compressible than the a- and c-axes. The compound also displays semiconductor-like behavior at high pressures, indicating potential applications in electronic devices .
Properties Under High Pressure:
- Compressibility: Anisotropic behavior with varying compressibility along different crystallographic axes.
- Electronic Transition: Tendency to behave as a semiconductor under high pressures.
- Optical Properties: Changes in refractive index and absorption spectra with pressure variations.
Synthesis of Azide Derivatives
This compound is utilized in the synthesis of various azide derivatives through lithiation reactions. This process involves converting lithiated intermediates into azides using reagents such as tosyl azide. The resulting compounds have applications in organic synthesis and materials science, particularly in the development of functional polymers .
Synthesis Process:
- Lithiation Reaction: Utilizes n-butyllithium to create lithiated intermediates.
- Conversion to Azides: Treatment with tosyl azide yields functionalized products.
- Applications: Useful in creating polymers with azide functionalities for further chemical modifications.
Thermal Decomposition Studies
The thermal stability and decomposition behavior of this compound have been extensively studied, revealing insights into its safety and handling properties. Research indicates that this compound decomposes at specific temperatures, producing nitrogen gas and other byproducts, which is crucial for applications where thermal stability is essential .
Decomposition Characteristics:
- Thermal Stability: Decomposition rates vary based on preparation methods.
- Safety Considerations: Understanding thermal behavior is critical for safe handling in industrial applications.
Potential Applications Beyond Energy Storage
Beyond its role in batteries, this compound shows potential in various advanced materials applications, including:
Mechanism of Action
The mechanism of action of lithium azide primarily involves its decomposition into lithium and nitrogen gas. This decomposition is highly exothermic and can be triggered by heat or shock. The azide ion (N3-) acts as a nucleophile in various chemical reactions, facilitating the formation of new bonds and the generation of nitrogen gas. The molecular targets and pathways involved in these reactions depend on the specific reagents and conditions used .
Comparison with Similar Compounds
Lithium azide belongs to the broader class of metal azides, which share the N₃⁻ anion but differ in their cation size, stability, and reactivity. Below is a detailed comparison with structurally analogous alkali metal azides (sodium, potassium, rubidium, cesium) and functionally similar lead azide.
Structural Analogues: Alkali Metal Azides
Alkali metal azides (MN₃, where M = Li, Na, K, Rb, Cs) exhibit systematic trends in stability and decomposition behavior due to cation size and electronegativity differences.
- Thermal Stability : Larger cations (e.g., Cs⁺) stabilize the azide ion through weaker cation-anion interactions, resulting in higher decomposition temperatures. This compound, with its small cation, decomposes explosively at lower temperatures (~150–200°C) compared to NaN₃ (~275°C) and CsN₃ (~390°C) .
- Reactivity : this compound reacts violently with water and acids, releasing toxic hydrazoic acid (HN₃). Sodium azide is less moisture-sensitive but reacts similarly with acids .
- Synthesis : NaN₃ is industrially produced via the "Wislicenus process" (ammonia + sodium amide + nitrous oxide), whereas LiN₃ requires metathesis due to lithium’s high hydration energy .
Functional Analogue: Lead Azide (Pb(N₃)₂)
Lead azide, a primary explosive, contrasts with this compound in both structure and application:
- Electronic Properties : Ab initio studies reveal that lead azide’s covalent bonding and band structure contribute to its detonation propensity, whereas LiN₃’s ionic bonds result in lower energy release .
- Applications : Lead azide is critical in detonators due to its reliable initiation, while this compound’s instability confines it to laboratory settings .
Comparison with Lithium Nitride (Li₃N)
- Phase Behavior : Li₃N undergoes pressure-induced phase transitions, making it valuable for energy storage, whereas LiN₃ lacks such utility due to decomposition .
Biological Activity
Lithium azide (LiN₃) is a compound with notable chemical properties and applications, particularly in the fields of chemistry and materials science. However, its biological activity is less commonly explored but presents interesting implications, especially in pharmacology and electrochemistry. This article reviews the biological activity of this compound, focusing on its mechanisms of action, potential applications in medicine, and relevant research findings.
This compound is the lithium salt of hydrazoic acid and is characterized by its ability to decompose into lithium and nitrogen gas upon heating. This decomposition reaction is significant as it influences the compound's interactions in biological systems:
-
Decomposition Reaction :
This reaction can occur at elevated temperatures (160° - 220°C) and is crucial for understanding its stability and reactivity in various environments .
-
Biochemical Pathways :
Lithium ions are known to affect several neurotransmitter systems, particularly those involving dopamine and glutamate, which are critical in mood regulation and psychiatric conditions such as bipolar disorder. The presence of lithium in this compound suggests potential therapeutic effects similar to those observed with lithium carbonate.
Case Studies
- Thermal Decomposition Studies : Research has shown that the thermal decomposition kinetics of this compound vary significantly based on its physical form (powder vs. pellet). Studies indicated that pre-irradiation with gamma or UV radiation alters the decomposition behavior, suggesting potential applications in controlled reactions within biological systems .
- Electrochemical Properties : In a study investigating this compound as an electrolyte additive, it was found to improve cycling performance significantly compared to traditional additives like lithium nitrate. The formation of a compact passivation layer on the lithium anode was highlighted as a key mechanism enhancing ionic conductivity and overall battery efficiency .
Data Table: Comparative Analysis of this compound Applications
Q & A
Basic Research Questions
Q. What are the standard laboratory methods for synthesizing lithium azide, and how is purity validated?
this compound (LiN₃) is synthesized via metathesis reactions, such as reacting sodium azide (NaN₃) with lithium nitrate (LiNO₃) or lithium sulfate (Li₂SO₄) in aqueous solutions. For example, barium azide (Ba(N₃)₂) reacts with Li₂SO₄ to yield LiN₃ and BaSO₄ precipitate. Post-synthesis, purity is validated using:
- X-ray diffraction (XRD) : Confirms crystallinity and phase purity (e.g., Cu Kα radiation, 2θ range 10–80°).
- FTIR spectroscopy : Detects N₃⁻ asymmetric stretching modes (~2100 cm⁻¹).
- Elemental analysis : Verifies Li:N stoichiometry (target 1:3).
- Thermogravimetric analysis (TGA) : Determines decomposition onset (e.g., heating rate 10°C/min under N₂). Safety protocols (e.g., blast shields, fume hoods) are mandatory due to explosive risks .
Q. What safety protocols are essential for handling this compound in experimental settings?
- Use explosion-proof fume hoods and remote handling tools.
- Store solutions in HDPE containers at ≤4°C to slow decomposition.
- Avoid acid exposure (releases toxic HN₃ gas; R32 risk).
- Wear nitrile gloves, face shields, and lab coats.
- Neutralize spills with 10% sodium hypochlorite.
- Reference toxicity data (LD50) and MSDS sheets .
Q. How does this compound’s stability vary under different storage conditions?
LiN₃ decomposes at 115–298°C, with stability influenced by:
- Temperature : Storage below 4°C in anhydrous environments minimizes decomposition.
- pH : Neutral or slightly basic conditions prevent HN₃ formation.
- Light exposure : Opaque containers reduce photolytic degradation. Monitor stability via periodic TGA and FTIR to detect byproducts (e.g., LiOH, N₂) .
Advanced Research Questions
Q. How can density functional theory (DFT) simulations optimize modeling of this compound’s decomposition pathways?
- Software : Use Vienna Ab initio Simulation Package (VASP) with plane-wave basis sets and pseudopotentials.
- Parameters : Enforce energy convergence (≤1 meV/atom) and force thresholds (≤0.01 eV/Å).
- Methods : Apply nudged elastic band (NEB) for reaction coordinates and phonon analysis for transition states.
- Validation : Cross-check with experimental XRD and DSC data to resolve discrepancies .
Q. What combined experimental-computational approaches resolve contradictions in this compound’s electronic structure?
- Experimental : Angle-resolved photoemission spectroscopy (ARPES) and UV-Vis absorption for bandgap measurement.
- Computational : Hybrid DFT (HSE06) or GW approximations improve bandgap accuracy vs. standard DFT.
- Synchrotron techniques : X-ray absorption spectroscopy (XAS) probes unoccupied states.
- Error analysis : Document k-point convergence and pseudopotential selection to identify divergence sources .
Q. How do this compound’s decomposition mechanisms compare to sodium and lead azides?
- This compound : Decomposes exothermically at ~115°C, releasing N₂ gas.
- Sodium azide : Stable until ~300°C, decomposes into Na and N₂.
- Lead azide : Detonates at ~350°C due to Pb-N bond instability. Computational studies (e.g., DFT with van der Waals corrections) reveal differences in activation energies and lattice dynamics. Experimental validation via time-resolved mass spectrometry is critical .
Q. What methodological challenges arise in quantifying this compound’s reactivity with transition metals?
- Kinetic studies : Use stopped-flow spectroscopy to monitor rapid reactions (e.g., LiN₃ + Cu²⁺ → CuN₃).
- Byproduct analysis : Employ gas chromatography (GC) for N₂ quantification and ICP-MS for metal residues.
- Computational modeling : Combine DFT with molecular dynamics (MD) to simulate interfacial reactions. Address contradictions (e.g., unexpected intermediates) via in situ Raman spectroscopy .
Q. How do isotopic labeling studies enhance understanding of this compound’s nitrogen release kinetics?
- Labeling : Synthesize Li¹⁵N₃ to track nitrogen isotopologues via mass spectrometry.
- Kinetic models : Apply Arrhenius analysis to decomposition rates under controlled atmospheres.
- Data interpretation : Compare with computational predictions (e.g., transition state theory) to refine activation barriers. Discrepancies often arise from surface adsorption effects, requiring ultrahigh-vacuum (UHV) experimental setups .
Properties
IUPAC Name |
lithium;azide | |
---|---|---|
Source | PubChem | |
URL | https://pubchem.ncbi.nlm.nih.gov | |
Description | Data deposited in or computed by PubChem | |
InChI |
InChI=1S/Li.N3/c;1-3-2/q+1;-1 | |
Source | PubChem | |
URL | https://pubchem.ncbi.nlm.nih.gov | |
Description | Data deposited in or computed by PubChem | |
InChI Key |
GUWHRJQTTVADPB-UHFFFAOYSA-N | |
Source | PubChem | |
URL | https://pubchem.ncbi.nlm.nih.gov | |
Description | Data deposited in or computed by PubChem | |
Canonical SMILES |
[Li+].[N-]=[N+]=[N-] | |
Source | PubChem | |
URL | https://pubchem.ncbi.nlm.nih.gov | |
Description | Data deposited in or computed by PubChem | |
Molecular Formula |
LiN3 | |
Source | PubChem | |
URL | https://pubchem.ncbi.nlm.nih.gov | |
Description | Data deposited in or computed by PubChem | |
DSSTOX Substance ID |
DTXSID8066509 | |
Record name | Lithium azide (Li(N3)) | |
Source | EPA DSSTox | |
URL | https://comptox.epa.gov/dashboard/DTXSID8066509 | |
Description | DSSTox provides a high quality public chemistry resource for supporting improved predictive toxicology. | |
Molecular Weight |
49.0 g/mol | |
Source | PubChem | |
URL | https://pubchem.ncbi.nlm.nih.gov | |
Description | Data deposited in or computed by PubChem | |
CAS No. |
19597-69-4 | |
Record name | Lithium azide | |
Source | CAS Common Chemistry | |
URL | https://commonchemistry.cas.org/detail?cas_rn=19597-69-4 | |
Description | CAS Common Chemistry is an open community resource for accessing chemical information. Nearly 500,000 chemical substances from CAS REGISTRY cover areas of community interest, including common and frequently regulated chemicals, and those relevant to high school and undergraduate chemistry classes. This chemical information, curated by our expert scientists, is provided in alignment with our mission as a division of the American Chemical Society. | |
Explanation | The data from CAS Common Chemistry is provided under a CC-BY-NC 4.0 license, unless otherwise stated. | |
Record name | Lithium azide (Li(N3)) | |
Source | ChemIDplus | |
URL | https://pubchem.ncbi.nlm.nih.gov/substance/?source=chemidplus&sourceid=0019597694 | |
Description | ChemIDplus is a free, web search system that provides access to the structure and nomenclature authority files used for the identification of chemical substances cited in National Library of Medicine (NLM) databases, including the TOXNET system. | |
Record name | Lithium azide (Li(N3)) | |
Source | EPA Chemicals under the TSCA | |
URL | https://www.epa.gov/chemicals-under-tsca | |
Description | EPA Chemicals under the Toxic Substances Control Act (TSCA) collection contains information on chemicals and their regulations under TSCA, including non-confidential content from the TSCA Chemical Substance Inventory and Chemical Data Reporting. | |
Record name | Lithium azide (Li(N3)) | |
Source | EPA DSSTox | |
URL | https://comptox.epa.gov/dashboard/DTXSID8066509 | |
Description | DSSTox provides a high quality public chemistry resource for supporting improved predictive toxicology. | |
Record name | Lithium triazide | |
Source | European Chemicals Agency (ECHA) | |
URL | https://echa.europa.eu/substance-information/-/substanceinfo/100.039.237 | |
Description | The European Chemicals Agency (ECHA) is an agency of the European Union which is the driving force among regulatory authorities in implementing the EU's groundbreaking chemicals legislation for the benefit of human health and the environment as well as for innovation and competitiveness. | |
Explanation | Use of the information, documents and data from the ECHA website is subject to the terms and conditions of this Legal Notice, and subject to other binding limitations provided for under applicable law, the information, documents and data made available on the ECHA website may be reproduced, distributed and/or used, totally or in part, for non-commercial purposes provided that ECHA is acknowledged as the source: "Source: European Chemicals Agency, http://echa.europa.eu/". Such acknowledgement must be included in each copy of the material. ECHA permits and encourages organisations and individuals to create links to the ECHA website under the following cumulative conditions: Links can only be made to webpages that provide a link to the Legal Notice page. | |
Retrosynthesis Analysis
AI-Powered Synthesis Planning: Our tool employs the Template_relevance Pistachio, Template_relevance Bkms_metabolic, Template_relevance Pistachio_ringbreaker, Template_relevance Reaxys, Template_relevance Reaxys_biocatalysis model, leveraging a vast database of chemical reactions to predict feasible synthetic routes.
One-Step Synthesis Focus: Specifically designed for one-step synthesis, it provides concise and direct routes for your target compounds, streamlining the synthesis process.
Accurate Predictions: Utilizing the extensive PISTACHIO, BKMS_METABOLIC, PISTACHIO_RINGBREAKER, REAXYS, REAXYS_BIOCATALYSIS database, our tool offers high-accuracy predictions, reflecting the latest in chemical research and data.
Strategy Settings
Precursor scoring | Relevance Heuristic |
---|---|
Min. plausibility | 0.01 |
Model | Template_relevance |
Template Set | Pistachio/Bkms_metabolic/Pistachio_ringbreaker/Reaxys/Reaxys_biocatalysis |
Top-N result to add to graph | 6 |
Feasible Synthetic Routes
Disclaimer and Information on In-Vitro Research Products
Please be aware that all articles and product information presented on BenchChem are intended solely for informational purposes. The products available for purchase on BenchChem are specifically designed for in-vitro studies, which are conducted outside of living organisms. In-vitro studies, derived from the Latin term "in glass," involve experiments performed in controlled laboratory settings using cells or tissues. It is important to note that these products are not categorized as medicines or drugs, and they have not received approval from the FDA for the prevention, treatment, or cure of any medical condition, ailment, or disease. We must emphasize that any form of bodily introduction of these products into humans or animals is strictly prohibited by law. It is essential to adhere to these guidelines to ensure compliance with legal and ethical standards in research and experimentation.