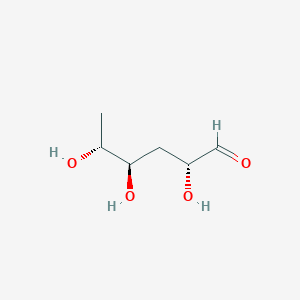
3,6-Dideoxy-D-xylo-hexose
Overview
Description
3,6-Dideoxy-D-xylo-hexose is an organic compound that belongs to the class of deoxy sugars. It is a six-carbon sugar, structurally similar to glucose, but with the hydroxyl groups at positions 3 and 6 replaced by hydrogen atoms. This compound is a colorless crystalline solid that is soluble in water and some organic solvents .
Mechanism of Action
Target of Action
3,6-Dideoxy-D-xylo-hexose, also known as Abequose , is a constituent of the O-specific chains in lipopolysaccharides that occur in certain serotypes of Salmonella and Citrobacter bacteria . These lipopolysaccharides are the primary targets of Abequose. They play a crucial role in maintaining the integrity of the outer membrane of Gram-negative bacteria .
Mode of Action
It is known that it interacts with its targets, the lipopolysaccharides, by becoming a part of their structure . This interaction may result in changes to the properties of the lipopolysaccharides, potentially affecting the functionality of the bacterial outer membrane.
Biochemical Pathways
Abequose is involved in the biosynthesis of lipopolysaccharides . The biosynthesis of hexose derivatives deoxygenated at C6 first requires the generation of a 6-deoxy-4-keto derivative intermediate catalyzed by a 4,6-dehydratase . This is followed by a combination of reduction, epimerization, and amino substitutions . These biochemical pathways lead to the formation of Abequose, which then becomes a part of the lipopolysaccharides.
Result of Action
The incorporation of Abequose into lipopolysaccharides could potentially affect the properties of these molecules, and by extension, the properties of the bacterial outer membrane. This could have various molecular and cellular effects, potentially influencing the bacteria’s survival and virulence .
Biochemical Analysis
Biochemical Properties
3,6-Dideoxy-D-xylo-hexose is involved in various biochemical reactions, particularly in the biosynthesis of bacterial lipopolysaccharides. It interacts with several enzymes, including CDP-6-deoxy-D-glucoseen reductase, which catalyzes the formation of CDP-3,6-dideoxy-D-xylo-hexose from CDP-4-keto-6-deoxy-D-glucose . This interaction is crucial for the incorporation of this compound into the lipopolysaccharide structure, contributing to the virulence and immune evasion mechanisms of pathogenic bacteria.
Cellular Effects
This compound affects various cellular processes, particularly in bacterial cells. It influences cell function by being a part of the lipopolysaccharide layer, which is essential for maintaining the integrity of the bacterial outer membrane . This compound can impact cell signaling pathways and gene expression by modulating the immune response of the host organism, thereby aiding in bacterial survival and pathogenicity.
Molecular Mechanism
At the molecular level, this compound exerts its effects through specific binding interactions with enzymes involved in its biosynthesis. The key enzyme, CDP-6-deoxy-D-glucoseen reductase, facilitates the reduction of CDP-4-keto-6-deoxy-D-glucose to form CDP-3,6-dideoxy-D-xylo-hexose . This reaction involves the transfer of electrons and protons, highlighting the enzyme’s role in the redox process. Additionally, this compound can influence gene expression by altering the structure and function of the lipopolysaccharide layer, which interacts with host immune receptors.
Temporal Effects in Laboratory Settings
In laboratory settings, the effects of this compound can change over time due to its stability and degradation properties. Studies have shown that this compound is relatively stable under standard laboratory conditions, but it can undergo degradation when exposed to extreme pH or temperature . Long-term effects on cellular function include alterations in the bacterial outer membrane’s structural integrity, which can impact bacterial viability and pathogenicity.
Dosage Effects in Animal Models
The effects of this compound vary with different dosages in animal models. At low doses, this compound can enhance bacterial survival by strengthening the lipopolysaccharide layer, while high doses may lead to toxic effects due to the overstimulation of the host immune response . Threshold effects observed in studies indicate that there is a critical concentration above which the compound’s beneficial effects are outweighed by its toxicity.
Metabolic Pathways
This compound is involved in the metabolic pathways of bacterial lipopolysaccharide biosynthesis. It is synthesized from CDP-4-keto-6-deoxy-D-glucose through the action of CDP-6-deoxy-D-glucoseen reductase . This pathway involves several enzymatic steps, including reduction, dehydration, and glycosylation, which are essential for the incorporation of this compound into the lipopolysaccharide structure.
Transport and Distribution
Within bacterial cells, this compound is transported and distributed as part of the lipopolysaccharide assembly process. It interacts with specific transporters and binding proteins that facilitate its incorporation into the lipopolysaccharide layer . This localization is crucial for the compound’s function in maintaining the structural integrity and immunogenic properties of the bacterial outer membrane.
Subcellular Localization
This compound is primarily localized in the outer membrane of bacterial cells as a component of the lipopolysaccharide layer . This subcellular localization is directed by specific targeting signals and post-translational modifications that ensure the proper assembly and function of the lipopolysaccharide structure. The presence of this compound in the outer membrane is essential for the bacteria’s ability to evade the host immune system and establish infection.
Preparation Methods
The synthesis of 3,6-Dideoxy-D-xylo-hexose can be achieved through several routes. One practical method involves the use of 1,2-O-propylidene acetal to protect the monosaccharides, glucose, and galactose. This protection allows for easy modification at both the 3 and 6 positions and facilitates the placement of different protecting groups on the hydroxyl groups at positions 2 and 4 . Another method involves the reduction of methyl 3,4-anhydro-6-O-p-toluenesulfonyl-α-D-galactopyranoside by super hydride, which simultaneously achieves deoxygenation at positions 3 and 6 .
Chemical Reactions Analysis
3,6-Dideoxy-D-xylo-hexose undergoes various chemical reactions, including:
Oxidation: This reaction typically involves the use of strong oxidizing agents such as ruthenium tetroxide.
Reduction: Reduction reactions can be carried out using reagents like sodium borohydride or lithium aluminum hydride.
Substitution: Halogenation reactions can be performed using sulfuryl chloride, followed by dechlorosulfation with sodium iodide.
The major products formed from these reactions depend on the specific reagents and conditions used. For example, oxidation with ruthenium tetroxide can yield carboxylic acids, while reduction with sodium borohydride can produce alcohols .
Scientific Research Applications
Role in Lipopolysaccharides
3,6-Dideoxy-D-xylo-hexose is found predominantly in the lipopolysaccharides (LPS) of gram-negative bacteria. These sugars serve as essential antigenic determinants, influencing the immune response. Specifically, four out of five known 3,6-dideoxyhexoses occur in various strains of Salmonella enterica, including abequose, tyvelose, paratose, and colitose. The presence of these sugars contributes to the structural diversity of LPS and their immunogenic properties .
Antigenic Determinants
The structural variations introduced by this compound within LPS can significantly affect how the immune system recognizes bacterial pathogens. This has implications for vaccine development and understanding bacterial pathogenesis .
Synthesis and Derivatives
Research has focused on synthesizing this compound for use in creating glycosylated compounds that can serve as potential therapeutics. For example, the synthesis of disaccharide determinants containing unique 3,6-dideoxyhexoses has been explored for their utility in biosensor studies aimed at detecting bacterial infections .
Modifications for Enhanced Reactivity
Studies have shown that deoxygenation at specific positions alters the reactivity profiles of sugar derivatives. For instance, modifications at the C-3 position can enhance reactivity compared to fully hydroxylated substrates, indicating potential pathways for developing more effective glycosylation reactions in synthetic organic chemistry .
Antibiotic Development
The exploration of this compound's role in antibiotic function is significant due to its involvement in natural products with antimicrobial properties. Understanding how these sugars influence receptor binding and efficacy can lead to the design of novel antibiotics that leverage their structural uniqueness .
Immunological Applications
Given its role as an antigenic determinant in gram-negative bacteria, this compound could be pivotal in developing vaccines that target specific bacterial strains or enhance immune responses against infections caused by these pathogens .
Case Study: Salmonella enterica
A study highlighted the genetic basis for the biosynthesis of 3,6-dideoxyhexoses in Salmonella enterica, emphasizing the gene clusters responsible for producing these sugars and their implications for bacterial virulence . The cloning of specific biosynthetic genes has opened avenues for further mechanistic studies that could inform vaccine development.
Case Study: Yersinia pseudotuberculosis
Research into Yersinia pseudotuberculosis has revealed polymorphisms associated with 3,6-dideoxyhexoses that could affect pathogenicity and immune evasion strategies employed by this bacterium . Understanding these variations may lead to targeted therapeutic strategies.
Comparison with Similar Compounds
3,6-Dideoxy-D-xylo-hexose is similar to other deoxy sugars such as paratose (3,6-dideoxy-D-ribo-hexose) and tyvelose (3,6-dideoxy-D-arabino-hexose). it is unique in its specific structural configuration and its role in the lipopolysaccharide O-antigen of certain bacterial serotypes . Other similar compounds include:
Paratose: Found in the lipopolysaccharide of Salmonella serogroup A.
Tyvelose: Found in the lipopolysaccharide of certain other bacterial strains.
These compounds share similar chemical properties but differ in their specific biological roles and structural configurations.
Biological Activity
3,6-Dideoxy-D-xylo-hexose, commonly referred to as Abequose , is a sugar that plays a significant role in the biological activities of various organisms, particularly bacteria. Its involvement in the biosynthesis of lipopolysaccharides (LPS) is critical for understanding its biological functions and potential applications in medicine and industry.
This compound is characterized by the absence of hydroxyl groups at the C-3 and C-6 positions of the hexose structure, which influences its biochemical properties and interactions with other molecules. This compound is integral to the structure of LPS found in the outer membrane of Gram-negative bacteria, contributing to their virulence and immune evasion mechanisms.
Target of Action
Abequose primarily targets bacterial lipopolysaccharides. It integrates into their structure, modifying their properties and affecting bacterial survival and virulence. The incorporation of Abequose into LPS can alter membrane integrity and influence interactions with host immune systems.
Biochemical Pathways
The biosynthesis of this compound involves several enzymatic reactions, predominantly catalyzed by CDP-6-deoxy-D-glucoseen reductase . This enzyme facilitates the conversion of CDP-4-keto-6-deoxy-D-glucose to CDP-3,6-dideoxy-D-xylo-hexose . The following table summarizes key enzymes involved in its biosynthesis:
Enzyme | Reaction |
---|---|
CDP-6-deoxy-D-glucoseen reductase | CDP-4-keto-6-deoxy-D-glucose → CDP-3,6-dideoxy-D-xylo-hexose |
Various glycosyltransferases | Incorporation into lipopolysaccharides |
Cellular Effects
Abequose influences various cellular processes within bacterial cells, particularly through its role in LPS. It is essential for maintaining the structural integrity of the bacterial outer membrane, which is crucial for cell protection against environmental stresses and host immune responses .
Dosage Effects in Animal Models
Research indicates that different dosages of Abequose can have varying effects on bacterial survival. Low doses may enhance bacterial resilience by fortifying the LPS layer, while high doses could provoke toxic responses due to overstimulation of host immune mechanisms .
Research Findings
Several studies have investigated the biological activity of this compound:
- Antimicrobial Properties : Abequose has been shown to exhibit antimicrobial effects against specific pathogens such as E. coli and Staphylococcus aureus, suggesting its potential as a therapeutic agent .
- Immunological Implications : The presence of Abequose in LPS can modulate immune responses. Studies indicate that it may act as an immunodominant sugar in certain bacterial strains, influencing serological responses .
- Toxicological Studies : High concentrations of Abequose have been linked to adverse effects on host cells due to excessive immune activation. This highlights the importance of dosage regulation in therapeutic applications .
Case Study 1: Antibacterial Activity
In a laboratory setting, a study demonstrated that this compound exhibited significant antibacterial activity with minimum inhibitory concentration (MIC) values indicating effectiveness against E. coli at concentrations as low as 62.5 µg/mL .
Case Study 2: Immunomodulatory Effects
Another investigation revealed that Abequose could modulate inflammatory responses in RAW 264.7 macrophages stimulated with lipopolysaccharides. At a concentration of 100 μg/mL, it inhibited nitric oxide release and altered cytokine expression profiles (e.g., IL-1β and TNF-α), demonstrating its potential as an anti-inflammatory agent .
Properties
IUPAC Name |
(3R,5R,6R)-6-methyloxane-2,3,5-triol | |
---|---|---|
Source | PubChem | |
URL | https://pubchem.ncbi.nlm.nih.gov | |
Description | Data deposited in or computed by PubChem | |
InChI |
InChI=1S/C6H12O4/c1-3-4(7)2-5(8)6(9)10-3/h3-9H,2H2,1H3/t3-,4-,5-,6?/m1/s1 | |
Source | PubChem | |
URL | https://pubchem.ncbi.nlm.nih.gov | |
Description | Data deposited in or computed by PubChem | |
InChI Key |
KYPWIZMAJMNPMJ-JDJSBBGDSA-N | |
Source | PubChem | |
URL | https://pubchem.ncbi.nlm.nih.gov | |
Description | Data deposited in or computed by PubChem | |
Canonical SMILES |
CC1C(CC(C(O1)O)O)O | |
Source | PubChem | |
URL | https://pubchem.ncbi.nlm.nih.gov | |
Description | Data deposited in or computed by PubChem | |
Isomeric SMILES |
C[C@@H]1[C@@H](C[C@H](C(O1)O)O)O | |
Source | PubChem | |
URL | https://pubchem.ncbi.nlm.nih.gov | |
Description | Data deposited in or computed by PubChem | |
Molecular Formula |
C6H12O4 | |
Source | PubChem | |
URL | https://pubchem.ncbi.nlm.nih.gov | |
Description | Data deposited in or computed by PubChem | |
Molecular Weight |
148.16 g/mol | |
Source | PubChem | |
URL | https://pubchem.ncbi.nlm.nih.gov | |
Description | Data deposited in or computed by PubChem | |
CAS No. |
644-48-4 | |
Record name | Abequose | |
Source | ChemIDplus | |
URL | https://pubchem.ncbi.nlm.nih.gov/substance/?source=chemidplus&sourceid=0000644484 | |
Description | ChemIDplus is a free, web search system that provides access to the structure and nomenclature authority files used for the identification of chemical substances cited in National Library of Medicine (NLM) databases, including the TOXNET system. | |
Retrosynthesis Analysis
AI-Powered Synthesis Planning: Our tool employs the Template_relevance Pistachio, Template_relevance Bkms_metabolic, Template_relevance Pistachio_ringbreaker, Template_relevance Reaxys, Template_relevance Reaxys_biocatalysis model, leveraging a vast database of chemical reactions to predict feasible synthetic routes.
One-Step Synthesis Focus: Specifically designed for one-step synthesis, it provides concise and direct routes for your target compounds, streamlining the synthesis process.
Accurate Predictions: Utilizing the extensive PISTACHIO, BKMS_METABOLIC, PISTACHIO_RINGBREAKER, REAXYS, REAXYS_BIOCATALYSIS database, our tool offers high-accuracy predictions, reflecting the latest in chemical research and data.
Strategy Settings
Precursor scoring | Relevance Heuristic |
---|---|
Min. plausibility | 0.01 |
Model | Template_relevance |
Template Set | Pistachio/Bkms_metabolic/Pistachio_ringbreaker/Reaxys/Reaxys_biocatalysis |
Top-N result to add to graph | 6 |
Feasible Synthetic Routes
Disclaimer and Information on In-Vitro Research Products
Please be aware that all articles and product information presented on BenchChem are intended solely for informational purposes. The products available for purchase on BenchChem are specifically designed for in-vitro studies, which are conducted outside of living organisms. In-vitro studies, derived from the Latin term "in glass," involve experiments performed in controlled laboratory settings using cells or tissues. It is important to note that these products are not categorized as medicines or drugs, and they have not received approval from the FDA for the prevention, treatment, or cure of any medical condition, ailment, or disease. We must emphasize that any form of bodily introduction of these products into humans or animals is strictly prohibited by law. It is essential to adhere to these guidelines to ensure compliance with legal and ethical standards in research and experimentation.