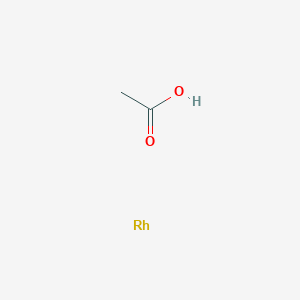
Acetic acid rhodium
- Click on QUICK INQUIRY to receive a quote from our team of experts.
- With the quality product at a COMPETITIVE price, you can focus more on your research.
Overview
Description
Acetic acid rhodium catalysts, particularly the anionic complex [Rh(CO)₂I₂]⁻, are central to the Monsanto process, a cornerstone of industrial acetic acid production via methanol carbonylation . This rhodium-based system operates under mild conditions (150–200°C, 30–60 bar CO) and achieves high selectivity (>95%) for acetic acid . The catalytic cycle involves oxidative addition of methyl iodide to Rh(I), followed by CO insertion and reductive elimination of acetyl iodide . However, the stability of the rhodium catalyst is highly dependent on water concentration; low water levels (<10 wt.%) lead to deactivation through dimerization or precipitation of Rh(III) species (e.g., trans-[Rh(CO)₂I₄]⁻) .
Preparation Methods
Synthetic Routes and Reaction Conditions
Rhodium acetate is typically prepared by heating hydrated rhodium(III) chloride in a mixture of methanol and acetic acid. The reaction conditions involve:
Temperature: Elevated temperatures to facilitate the reaction.
Solvents: Methanol and acetic acid.
Reaction Time: Sufficient time to allow the formation of the bis(methanol) complex, which is then desolvated to yield rhodium acetate.
Industrial Production Methods
In industrial settings, the production of rhodium acetate follows similar principles but on a larger scale. The process involves:
Reactors: Large-scale reactors to handle the increased volume of reactants.
Purification: Techniques such as crystallization and filtration to purify the final product.
Quality Control: Ensuring the product meets specific purity standards for its intended applications.
Chemical Reactions Analysis
Types of Reactions
Rhodium acetate undergoes various types of chemical reactions, including:
Oxidation: It can act as an oxidizing agent in certain reactions.
Reduction: It can also be reduced under specific conditions.
Substitution: The acetate groups can be substituted with other ligands.
Common Reagents and Conditions
Oxidation: Common oxidizing agents include oxygen and peroxides.
Reduction: Reducing agents such as hydrogen gas or hydrides.
Substitution: Ligands such as phosphines or amines can replace the acetate groups.
Major Products Formed
Oxidation: Products include oxidized organic compounds.
Reduction: Reduced forms of rhodium complexes.
Substitution: New rhodium complexes with different ligands.
Scientific Research Applications
Carbonylation Reactions
One of the most notable applications of acetic acid rhodium complexes is in carbonylation reactions. The Monsanto process , a key industrial method for producing acetic acid from methanol and carbon monoxide, employs rhodium-based catalysts. This process has been essential for large-scale acetic acid production and highlights the efficiency of rhodium in facilitating the reaction:Methanol+Carbon MonoxideRhodium CatalystAcetic AcidThe efficiency of this process has led to its widespread adoption in the chemical industry .
Hydroformylation
Rhodium catalysts are also pivotal in hydroformylation reactions, which convert alkenes into aldehydes. This reaction is crucial for producing various chemicals used in detergents, fragrances, and pharmaceuticals. In this context, this compound complexes can enhance the selectivity and yield of the desired aldehyde products .
Production of Acetic Anhydride
Acetic anhydride is another important product derived from acetic acid through rhodium-catalyzed carbonylation processes. Acetic anhydride is widely used in pharmaceuticals, dyestuffs, and agricultural chemicals. The synthesis involves the use of this compound complexes to facilitate the reaction between methyl acetate and carbon monoxide .
Application | Description |
---|---|
Carbonylation | Conversion of methanol and CO to produce acetic acid |
Hydroformylation | Conversion of alkenes to aldehydes |
Production of Acetic Anhydride | Synthesis from methyl acetate using rhodium catalysts |
Industrial Case Study: Monsanto Process
The Monsanto process exemplifies the practical application of this compound complexes in industry. This process has revolutionized acetic acid production by significantly improving yield and reducing by-products compared to traditional methods. The use of a rhodium catalyst allows for lower reaction temperatures and pressures, contributing to energy efficiency .
Research Case Study: Hydroformylation Advances
Recent research has demonstrated advancements in hydroformylation using this compound complexes. Studies have shown that modifying the ligand environment around the rhodium can enhance catalytic activity and selectivity towards specific aldehyde products. This research opens avenues for developing more efficient catalytic systems for industrial applications .
Mechanism of Action
Rhodium acetate exerts its effects through its ability to coordinate with various ligands and catalyze chemical reactions. The mechanism involves:
Molecular Targets: Organic substrates that undergo transformation in the presence of rhodium acetate.
Pathways Involved: Catalytic cycles that facilitate the conversion of reactants to products.
Comparison with Similar Compounds
Comparison with Iridium-Based Catalysts (Cativa Process)
The Cativa process, commercialized in 1995, employs an iridium catalyst (e.g., [Ir(CO)₂I₂]⁻) with a ruthenium promoter. Key comparisons include:
The Cativa process improves energy efficiency by reducing water content, lowering separation costs, but requires higher CO pressure (40–60 bar) .
Bimetallic Rhodium/Ruthenium Catalysts
A Rh(I)/Ru(III) bimetallic catalyst demonstrates enhanced performance:
- Conversion/Yield : 96% methyl acetate conversion with 96% acetic acid yield under optimized conditions .
- Stability: No precipitate formation and reduced water requirements due to electronic synergy. Ru modifies Rh’s electron density, accelerating methyl iodide oxidative addition .
- Mechanism: The bridged dimer structure minimizes steric hindrance, improving CO coordination and turnover frequency .
Cobalt and Nickel Catalytic Systems
- Cobalt (BASF Process) :
- Nickel: Limited activity; requires aggressive promoters (e.g., HI). Prone to corrosion and rapid deactivation .
Palladium and Other Metal Catalysts
- Palladium: Pd(OAc)₂-HI-PPh₃ systems show moderate activity (175°C, 50 bar CO) but lower turnover rates than Rh . Stability issues due to Pd aggregation under prolonged use.
- Gold: NHC-Au(I) catalysts enable ethane-to-acetic acid oxidation (40% yield) under mild conditions (80°C, 1 bar O₂) . Not directly comparable to carbonylation processes but offers an alternative route .
Structural and Electronic Modifications in Rhodium Catalysts
- Single-Atom Rh Catalysts: Rh₁O₅ sites in ZSM-5 micropores achieve 226.1 µmol/hr total products (acetic acid, methanol) with 0.10 wt% loading .
- Ligand Effects :
Economic and Industrial Considerations
- Market Dominance : Rh/Ir systems account for >90% of global acetic acid production due to high efficiency .
- Cost Challenges : Rhodium’s scarcity drives research into bimetallic or single-atom systems to reduce metal loading .
- Environmental Impact : Rh-based processes generate less waste than cobalt systems but require water management for stability .
Data Table: Comparative Performance of Catalysts
Biological Activity
Acetic acid rhodium complexes, particularly those involving rhodium(II) and rhodium(III), have garnered attention in the field of biological chemistry due to their unique structural and reactive properties. This article delves into the biological activity of this compound, focusing on its interactions with biological systems, potential therapeutic applications, and mechanisms of action.
Overview of Rhodium in Biological Systems
Rhodium is a rare transition metal with no known natural biological function. However, its complexes have been utilized in various biological applications due to their ability to interact with biomolecules, particularly proteins and nucleic acids. The biological activity of rhodium compounds is largely attributed to their coordination chemistry and the ability to form stable complexes with biomolecular targets .
- DNA Binding : Rhodium complexes, especially those with dipyridylamine ligands, have been shown to bind selectively to DNA mismatches. This property is particularly useful in targeting cells deficient in the mismatch repair (MMR) pathway, which is often associated with cancer resistance to conventional therapies . The binding affinity and specificity of these complexes make them promising candidates for targeted cancer therapies.
- Cellular Uptake and Cytotoxicity : Studies indicate that rhodium metalloinsertors exhibit accelerated cellular uptake, leading to selective cytotoxicity against MMR-deficient cell lines. This selectivity is crucial for minimizing damage to healthy cells while effectively targeting cancerous tissues .
- Induction of Necrosis : The interaction between rhodium complexes and cellular components can disrupt normal cellular functions, leading to necrosis in targeted cells. This mechanism has been observed in various studies where rhodium compounds were used as anti-cancer agents .
Case Studies
-
Case Study 1: Rhodium(II) Complexes as Antitumor Agents
Research demonstrated that specific rhodium(II) complexes could induce cell cycle disruption and necrosis in MMR-deficient cancer cells. These findings suggest a potential therapeutic role for rhodium-based drugs in treating certain types of cancer resistant to traditional chemotherapies . -
Case Study 2: Protein Interactions
Rhodium complexes have been shown to interact with proteins such as casein. These interactions can lead to the formation of stable protein-rhodium complexes that may alter protein function or stability, providing insights into their potential use as biochemical probes or therapeutic agents .
Data Table: Biological Activity Summary
Compound Type | Biological Target | Mechanism of Action | Selectivity |
---|---|---|---|
Rhodium(II) Complexes | DNA Mismatches | High-affinity binding | MMR-deficient cells |
Rhodium(III) Complexes | Proteins (e.g., casein) | Structural modification | Various proteins |
Acetic Acid-Rhodium | Cancer Cells | Induction of necrosis | MMR-deficient cells |
Q & A
Basic Research Questions
Q. How should researchers design experiments to study the catalytic efficiency of rhodium complexes in acetic acid synthesis?
- Methodology :
- Variable Control : Design experiments with controlled variables such as rhodium concentration, reaction temperature (typically 150–200°C), CO partial pressure, and promoter (e.g., methyl iodide) concentration, as these directly influence reaction kinetics .
- Kinetic Analysis : Use time-resolved sampling to measure reaction rates. Rhodium-catalyzed methanol carbonylation exhibits zero-order dependence on methanol and CO but first-order dependence on methyl iodide and rhodium concentration .
- Data Validation : Compare results with established kinetic models (e.g., Monsanto or Cativa processes) to validate mechanistic assumptions .
Q. What characterization techniques are critical for identifying rhodium species in heterogeneous catalysts?
- Methodology :
- Spectroscopy : Employ X-ray absorption spectroscopy (XAS) to probe rhodium oxidation states and coordination environments. Single-atom Rh catalysts in zeolites require high-resolution techniques like EXAFS to confirm isolated Rh sites .
- Electron Microscopy : Use TEM/STEM to visualize Rh dispersion on supports (e.g., ZSM-5 or carbon).
- Surface Analysis : XPS quantifies Rh surface composition and detects potential leaching during catalytic cycles .
Q. How can researchers optimize reaction conditions for rhodium-catalyzed acetic acid production while minimizing metal loss?
- Methodology :
- Stabilizer Screening : Test metal salt stabilizers (e.g., Ru or Sn salts) at molar ratios of 0.1:1 to 20:1 (metal:Rh) to inhibit Rh precipitation in low-water systems .
- Solvent Effects : Evaluate polar solvents (e.g., acetic acid) to enhance reaction rates and stabilize intermediates .
- Reusability Tests : Conduct multiple catalytic cycles with ICP-MS analysis to quantify Rh leaching and assess catalyst longevity .
Advanced Research Questions
Q. How can contradictory data on reaction mechanisms in rhodium-catalyzed carbonylation be resolved?
- Methodology :
- Isotopic Labeling : Use ¹³C-labeled CO or CH₃OH to trace carbon pathways and distinguish between competing mechanisms (e.g., methyl migration vs. CO insertion) .
- In-situ Spectroscopy : Operando IR or Raman spectroscopy monitors intermediate species (e.g., Rh–CO or Rh–CH₃) under realistic reaction conditions .
- Computational Modeling : Density functional theory (DFT) simulations clarify transition states and energetics, reconciling discrepancies between experimental and theoretical data .
Q. What advanced strategies enhance the stability of single-atom rhodium catalysts in oxidative methane conversion to acetic acid?
- Methodology :
- Support Engineering : Functionalize zeolite supports with nitrogen-rich polymers to anchor Rh atoms, preventing aggregation during pyrolysis .
- Synchrotron Studies : Utilize XAS and XPS at synchrotron facilities to track Rh structural changes during catalysis at atomic resolution .
- Accelerated Stability Testing : Expose catalysts to extreme conditions (e.g., high O₂ pressure) and use TEM to assess Rh mobility .
Q. How do promoter additives (e.g., iodide salts) influence the electronic structure of rhodium catalysts?
- Methodology :
- Electrochemical Analysis : Cyclic voltammetry reveals shifts in Rh redox potentials induced by iodide coordination .
- DFT Calculations : Model electron density changes in Rh complexes with/without promoters to predict ligand effects on catalytic activity .
- Microkinetic Modeling : Integrate experimental rate data with electronic structure insights to refine promoter-optimization strategies .
Q. Data Analysis & Reporting Guidelines
Q. What statistical approaches are recommended for analyzing catalytic performance data?
- Methodology :
- Multivariate Regression : Correlate reaction variables (temperature, pressure, Rh loading) with acetic acid yield using software like R or Python .
- Error Analysis : Report confidence intervals for kinetic parameters (e.g., turnover frequency) to quantify reproducibility .
- Data Archiving : Use platforms like Zenodo to share raw datasets, ensuring transparency and enabling meta-analyses .
Q. How should researchers structure manuscripts to meet journal standards for rhodium catalysis studies?
- Methodology :
- Abstract Clarity : Include background, purpose, methods (e.g., XAS, kinetic modeling), key findings (e.g., Rh stability trends), and implications .
- Reproducibility : Detail catalyst synthesis steps, reaction conditions, and characterization protocols to enable replication .
- Ethical Reporting : Disclose funding sources, conflicts of interest, and data availability per CONSORT or SRQR guidelines .
Properties
Molecular Formula |
C2H4O2Rh |
---|---|
Molecular Weight |
162.96 g/mol |
IUPAC Name |
acetic acid;rhodium |
InChI |
InChI=1S/C2H4O2.Rh/c1-2(3)4;/h1H3,(H,3,4); |
InChI Key |
NENDHUHGFRLXEN-UHFFFAOYSA-N |
Canonical SMILES |
CC(=O)O.[Rh] |
Related CAS |
5503-41-3 42204-14-8 |
Origin of Product |
United States |
Disclaimer and Information on In-Vitro Research Products
Please be aware that all articles and product information presented on BenchChem are intended solely for informational purposes. The products available for purchase on BenchChem are specifically designed for in-vitro studies, which are conducted outside of living organisms. In-vitro studies, derived from the Latin term "in glass," involve experiments performed in controlled laboratory settings using cells or tissues. It is important to note that these products are not categorized as medicines or drugs, and they have not received approval from the FDA for the prevention, treatment, or cure of any medical condition, ailment, or disease. We must emphasize that any form of bodily introduction of these products into humans or animals is strictly prohibited by law. It is essential to adhere to these guidelines to ensure compliance with legal and ethical standards in research and experimentation.