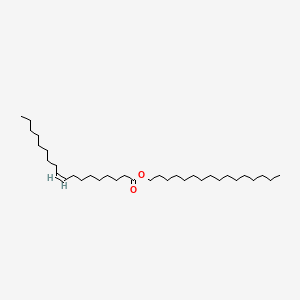
Palmityl oleate
Overview
Description
Palmityl oleate (C34H66O2; CAS 22393-86-8) is a wax ester synthesized via the esterification of hexadecan-1-ol (C16:0 alcohol) and oleic acid (C18:1 fatty acid) . It is characterized by a monounsaturated acyl chain, contributing to its lower melting temperature (Tm) compared to fully saturated analogs. With a molecular weight of 506.89 g/mol, it is a solid at room temperature and stored under frozen conditions for stability .
This compound is utilized in diverse applications:
- Biomedical Research: Serves as a model lipid for studying human meibomian gland secretions (tear film lipids) due to its structural similarity to natural wax esters .
- Cosmetics: Used to screen interactions between eyeliner cosmetics and lipid phases .
- Microbial Biotechnology: Produced by engineered bacteria (e.g., Acinetobacter sp.) in the presence of oleate and alcohols .
Preparation Methods
Chemical Synthesis Pathways
Acid-Catalyzed Esterification
Conventional acid-catalyzed esterification remains the most widely used method for palmityl oleate synthesis. In this approach, palmitic acid reacts with oleyl alcohol in the presence of acidic catalysts such as sulfuric acid (H₂SO₄), p-toluenesulfonic acid (PTSA), or sodium bisulfate (NaHSO₄). For instance, NaHSO₄ demonstrated superior catalytic efficiency in esterifying oleic acid with oleyl alcohol, achieving a 96.8% yield under optimized conditions (130°C, 9.9 wt% catalyst, 8-hour reaction) . Similarly, PTSA in toluene facilitated ricinoleic acid esterification at 70–80°C, with post-reaction purification involving water washes and magnesium sulfate drying .
Key Variables:
-
Catalyst Load: NaHSO₄ at 9.9 wt% relative to palmitic acid maximized yield while minimizing side reactions .
-
Temperature: Elevated temperatures (110–130°C) accelerated reaction kinetics but risked thermal degradation of oleyl alcohol .
-
Molar Ratio: A 1:1 molar ratio of palmitic acid to oleyl alcohol minimized unreacted substrates .
Table 1: Comparative Performance of Acid Catalysts
Enzymatic Synthesis
Lipases, particularly lipoprotein lipase (LPL), enable eco-friendly this compound synthesis under ambient conditions. LPL catalyzed the reaction between emulsified oleic acid and hexadecanol, producing this compound in a time- and dose-dependent manner . The absence of solvent and energy-intensive steps makes this method industrially appealing, though enzyme costs and scalability challenges persist.
Optimization Insights:
-
Emulsification: Gum arabic stabilized the oleic acid-hexadecanol emulsion, enhancing enzyme-substrate interaction .
-
Enzyme Load: Proportional increases in LPL concentration correlated linearly with yield up to saturation .
Reaction Optimization and Kinetic Studies
Temperature and Time Dependence
A study on oleyl oleate synthesis revealed that yield plateaued after 8 hours at 130°C, beyond which side reactions dominated . Similarly, enzymatic reactions achieved maximal conversion within 7–10 hours at 30–35°C .
Solvent Systems
Solvent-free conditions favored industrial scalability, while organic solvents like acetone improved homogeneity in small-scale reactions . For instance, acetone increased 1,2-diglyceride yields by 15% in palm oil intermediate fractionation .
Table 2: Impact of Solvent on Yield
Industrial-Scale Production Considerations
Catalyst Recovery and Reusability
NaHSO₄’s low cost and ease of separation via filtration make it preferable for continuous processes . In contrast, enzymatic methods require immobilized lipases to enable reuse, though carrier costs remain prohibitive for large-scale applications .
Purification Techniques
Molecular distillation (175°C, 0.8 Pa) effectively separated unreacted fatty acids and partial glycerides from crude this compound . Ethyl acetate extraction followed by NaHCO₃ neutralization further purified the product .
Comparative Analysis of Synthesis Routes
Table 3: Method Comparison
Parameter | Acid-Catalyzed | Enzymatic |
---|---|---|
Yield (%) | 85–97 | 70–85 |
Temperature (°C) | 70–130 | 20–40 |
Catalyst Cost | Low | High |
Environmental Impact | Moderate | Low |
Acid-catalyzed methods dominate due to higher yields and lower costs, while enzymatic routes appeal to green chemistry initiatives despite moderate efficiency .
Chemical Reactions Analysis
Types of Reactions: Palmityl oleate undergoes various chemical reactions, including:
Oxidation: It can be oxidized to form different products, depending on the conditions and reagents used.
Reduction: Reduction reactions can convert this compound into other derivatives.
Substitution: It can undergo substitution reactions where the ester group is replaced by other functional groups.
Common Reagents and Conditions:
Oxidation: Common oxidizing agents include potassium permanganate and hydrogen peroxide.
Reduction: Reducing agents such as lithium aluminum hydride can be used.
Substitution: Acidic or basic conditions can facilitate substitution reactions.
Major Products Formed: The major products formed from these reactions depend on the specific reagents and conditions used. For example, oxidation can yield carboxylic acids, while reduction can produce alcohols .
Scientific Research Applications
Palmityl oleate has a wide range of applications in scientific research:
Mechanism of Action
The mechanism of action of palmityl oleate involves its interaction with cellular membranes and proteins. It can modulate membrane fluidity and influence protein function through processes such as palmitoylation. Palmitoylation is a reversible lipid modification where a palmitoyl group is covalently attached to cysteine residues on proteins, affecting their localization, stability, and interactions .
Comparison with Similar Compounds
Comparison with Similar Wax Esters
Structural and Thermal Properties
Wax esters are classified by their alcohol (R-OH) and fatty acid (R'-COOH) components. Key structural variations include chain length, saturation, and branching. Palmityl oleate’s properties are compared below with saturated, unsaturated, and branched analogs:
Table 1: Thermal and Structural Properties of Selected Wax Esters
Key Findings :
- Saturation : Fully saturated esters (e.g., palmityl palmitate) exhibit higher Tm due to tighter molecular packing. This compound’s Tm (18.5°C) is ~20°C lower than palmityl palmitate .
- Chain Length : Longer acyl chains (e.g., behenyl oleate, C22:0/C18:1) increase Tm by 18.4°C compared to this compound .
- Mixtures : Binary mixtures (e.g., this compound + behenyl oleate) exhibit bimodal phase transitions, with Tm lower than individual components. Complex mixtures (≥4 components) form broad, asymmetric melting profiles .
Chromatographic Behavior
Unsaturated wax esters elute earlier than saturated analogs in gas chromatography due to reduced hydrophobicity. For example:
Biological Activity
Palmityl oleate, a wax ester formed from the condensation of hexadecan-1-ol (palmitol) and oleic acid, is a compound of interest due to its potential biological activities. This article delves into the biological activity of this compound, focusing on its interactions with cellular processes, particularly in the context of insulin resistance, mitochondrial function, and inflammation.
Chemical Structure and Properties
This compound is represented by the chemical formula and is classified as a wax ester. Its structure is significant because it combines a saturated fatty acid (palmitic acid) with an unsaturated fatty acid (oleic acid), which may influence its biological effects.
Insulin Resistance and Inflammation
Research indicates that this compound may play a protective role against palmitate-induced insulin resistance (IR). Palmitate, a saturated fatty acid, has been shown to induce IR and apoptosis in skeletal muscle cells. In contrast, oleate has been found to improve insulin sensitivity and prevent apoptosis. Co-incubation of palmitate with oleate reduces inflammation and improves mitochondrial function by promoting triglyceride accumulation and enhancing mitochondrial β-oxidation through PPARγ-dependent mechanisms .
Table 1: Effects of Fatty Acids on Insulin Resistance
Fatty Acid | Effect on Insulin Sensitivity | Induces Apoptosis | Mechanism |
---|---|---|---|
Palmitate | Decreases | Yes | Increases mtROS, diacylglycerol accumulation |
Oleate | Increases | No | Enhances mitochondrial function, reduces inflammation |
Mitochondrial Function
The role of this compound in mitochondrial function is particularly noteworthy. Studies have shown that while palmitate increases mitochondrial reactive oxygen species (mtROS) production leading to oxidative stress and damage, oleate does not induce mtROS generation and can ameliorate the damaging effects of palmitate. The addition of oleate has been shown to restore mitochondrial function by increasing ATP levels and preventing palmitate-induced apoptosis .
Case Study: Mitochondrial Dysfunction
In a controlled laboratory study, L6 skeletal muscle cells were treated with varying concentrations of palmitate and oleate. The results demonstrated that:
- Palmitate treatment led to significant mtDNA damage and increased apoptosis.
- Co-treatment with oleate resulted in reduced mtDNA damage and increased cell viability, highlighting oleate's protective effects against the deleterious impacts of palmitate .
Implications for Health
The contrasting effects of saturated versus unsaturated fatty acids like this compound underscore their significance in metabolic health. The ability of oleate to mitigate the harmful effects of palmitate suggests potential therapeutic applications in conditions characterized by insulin resistance, such as type 2 diabetes and obesity.
Q & A
Basic Research Questions
Q. How is palmityl oleate synthesized and characterized in laboratory settings?
this compound is synthesized via esterification of palmitic acid and oleyl alcohol, typically catalyzed by acid or enzymatic agents. Characterization involves nuclear magnetic resonance (NMR) for structural confirmation, gas chromatography (GC) or high-performance liquid chromatography (HPLC) for purity assessment, and mass spectrometry (MS) for molecular weight verification. For reproducibility, experimental protocols should detail reaction conditions (e.g., molar ratios, temperature, solvent systems) and purification steps (e.g., column chromatography). Known compounds require cross-referencing with literature data, while novel derivatives demand elemental analysis and spectral comparisons .
Q. What analytical methods are recommended for assessing this compound purity and lipid composition?
Purity analysis relies on chromatographic techniques (GC or HPLC) coupled with flame ionization or mass spectrometric detection. Fatty acid composition is determined using saponification followed by derivatization to fatty acid methyl esters (FAMEs), analyzed via GC with reference to standardized profiles (e.g., USP 〈401〉). Impurity testing includes heavy metal quantification (Method II 〈231〉) and alkaline impurity titration using bromophenol blue as an indicator .
Q. What are the key thermal properties of this compound, and how are they measured experimentally?
Differential scanning calorimetry (DSC) is the primary method for determining melting temperature (), enthalpy change (), and cooperative unit size. For reproducible results, use standardized "dry loading" procedures to minimize moisture interference. Published values vary due to differences in experimental protocols (e.g., heating rates, sample preparation), so researchers should report instrument-specific parameters (e.g., 2–10°C/min heating rate) and validate against internal controls .
Advanced Research Questions
Q. How can contradictions in published thermal data for this compound be resolved?
Discrepancies in values often arise from methodological variations (e.g., "clearing point" vs. measurements) or batch-specific impurities. To address this:
- Compare DSC protocols (e.g., heating rate, sample mass) across studies.
- Validate results using multiple analytical techniques (e.g., X-ray diffraction for crystallinity).
- Replicate experiments using identical purification and calibration standards. Critical evaluation of historical data should note the absence of universal calibration practices in lipid thermal analysis .
Q. What experimental design considerations are critical for studying this compound’s interactions in lipid matrices?
Use a PICOT framework to structure the research question:
- P opulation: Lipid system (e.g., meibomian secretions, synthetic bilayers).
- I ntervention: Incorporation of this compound at varying concentrations.
- C omparison: Baseline lipid profiles without this compound.
- O utcome: Phase behavior (e.g., shifts, enthalpy changes).
- T ime: Duration of thermal cycling or equilibration. Combine DSC with Langmuir monolayer assays to assess interfacial behavior and cooperativity .
Q. How can lipid extraction protocols (e.g., Bligh-Dyer) be optimized for this compound recovery from heterogeneous biological samples?
The Bligh-Dyer method (chloroform:methanol:water, 2:1:0.8 v/v) efficiently extracts this compound but may require adjustments for tissues with high phospholipid content:
- Increase chloroform ratios for hydrophobic matrices.
- Centrifuge at 4°C to prevent lipid degradation.
- Validate recovery rates using spiked internal standards (e.g., deuterated analogs) and GC-MS quantification .
Q. What statistical approaches ensure reliability in this compound quantification across subsamples?
Apply error propagation models to account for sampling heterogeneity:
- Calculate sampling errors () using incremental subsampling (≥10 increments per sample).
- Use ANOVA to assess inter-batch variability.
- Report confidence intervals (95%) for analytical precision. Documentation must include subsampling equipment, homogenization steps, and pre-analysis storage conditions to ensure traceability .
Q. How can synthetic byproducts in this compound preparations be identified and mitigated?
Monitor byproducts (e.g., unreacted palmitic acid, transesterification products) via:
- Thin-layer chromatography (TLC) with iodine staining.
- Adjusting reaction stoichiometry and catalyst loading.
- Implementing post-synthesis purification (e.g., silica gel chromatography). Purity thresholds should align with USP guidelines for lipid excipients .
Methodological Best Practices
- Data Reporting : Follow journal-specific guidelines (e.g., Beilstein Journal of Organic Chemistry) for experimental replication, including detailed synthesis protocols and spectral datasets in supplementary materials .
- Instrument Calibration : Validate DSC and GC systems daily using certified reference materials (e.g., indium for DSC, FAME mixtures for GC).
- Ethical Compliance : Disclose conflicts of interest and obtain permissions for adapted methodologies .
Properties
IUPAC Name |
hexadecyl octadec-9-enoate | |
---|---|---|
Details | Computed by LexiChem 2.6.6 (PubChem release 2019.06.18) | |
Source | PubChem | |
URL | https://pubchem.ncbi.nlm.nih.gov | |
Description | Data deposited in or computed by PubChem | |
InChI |
InChI=1S/C34H66O2/c1-3-5-7-9-11-13-15-17-19-20-22-24-26-28-30-32-34(35)36-33-31-29-27-25-23-21-18-16-14-12-10-8-6-4-2/h17,19H,3-16,18,20-33H2,1-2H3 | |
Details | Computed by InChI 1.0.5 (PubChem release 2019.06.18) | |
Source | PubChem | |
URL | https://pubchem.ncbi.nlm.nih.gov | |
Description | Data deposited in or computed by PubChem | |
InChI Key |
JYTMDBGMUIAIQH-UHFFFAOYSA-N | |
Details | Computed by InChI 1.0.5 (PubChem release 2019.06.18) | |
Source | PubChem | |
URL | https://pubchem.ncbi.nlm.nih.gov | |
Description | Data deposited in or computed by PubChem | |
Canonical SMILES |
CCCCCCCCCCCCCCCCOC(=O)CCCCCCCC=CCCCCCCCC | |
Details | Computed by OEChem 2.1.5 (PubChem release 2019.06.18) | |
Source | PubChem | |
URL | https://pubchem.ncbi.nlm.nih.gov | |
Description | Data deposited in or computed by PubChem | |
Molecular Formula |
C34H66O2 | |
Details | Computed by PubChem 2.1 (PubChem release 2019.06.18) | |
Source | PubChem | |
URL | https://pubchem.ncbi.nlm.nih.gov | |
Description | Data deposited in or computed by PubChem | |
DSSTOX Substance ID |
DTXSID1066788 | |
Record name | 9-Octadecenoic acid (9Z)-, hexadecyl ester | |
Source | EPA DSSTox | |
URL | https://comptox.epa.gov/dashboard/DTXSID1066788 | |
Description | DSSTox provides a high quality public chemistry resource for supporting improved predictive toxicology. | |
Molecular Weight |
506.9 g/mol | |
Details | Computed by PubChem 2.1 (PubChem release 2021.05.07) | |
Source | PubChem | |
URL | https://pubchem.ncbi.nlm.nih.gov | |
Description | Data deposited in or computed by PubChem | |
CAS No. |
22393-86-8 | |
Record name | 9-Octadecenoic acid (9Z)-, hexadecyl ester | |
Source | EPA Chemicals under the TSCA | |
URL | https://www.epa.gov/chemicals-under-tsca | |
Description | EPA Chemicals under the Toxic Substances Control Act (TSCA) collection contains information on chemicals and their regulations under TSCA, including non-confidential content from the TSCA Chemical Substance Inventory and Chemical Data Reporting. | |
Record name | 9-Octadecenoic acid (9Z)-, hexadecyl ester | |
Source | EPA DSSTox | |
URL | https://comptox.epa.gov/dashboard/DTXSID1066788 | |
Description | DSSTox provides a high quality public chemistry resource for supporting improved predictive toxicology. | |
Record name | Hexadecyl oleate | |
Source | European Chemicals Agency (ECHA) | |
URL | https://echa.europa.eu/substance-information/-/substanceinfo/100.040.848 | |
Description | The European Chemicals Agency (ECHA) is an agency of the European Union which is the driving force among regulatory authorities in implementing the EU's groundbreaking chemicals legislation for the benefit of human health and the environment as well as for innovation and competitiveness. | |
Explanation | Use of the information, documents and data from the ECHA website is subject to the terms and conditions of this Legal Notice, and subject to other binding limitations provided for under applicable law, the information, documents and data made available on the ECHA website may be reproduced, distributed and/or used, totally or in part, for non-commercial purposes provided that ECHA is acknowledged as the source: "Source: European Chemicals Agency, http://echa.europa.eu/". Such acknowledgement must be included in each copy of the material. ECHA permits and encourages organisations and individuals to create links to the ECHA website under the following cumulative conditions: Links can only be made to webpages that provide a link to the Legal Notice page. | |
Retrosynthesis Analysis
AI-Powered Synthesis Planning: Our tool employs the Template_relevance Pistachio, Template_relevance Bkms_metabolic, Template_relevance Pistachio_ringbreaker, Template_relevance Reaxys, Template_relevance Reaxys_biocatalysis model, leveraging a vast database of chemical reactions to predict feasible synthetic routes.
One-Step Synthesis Focus: Specifically designed for one-step synthesis, it provides concise and direct routes for your target compounds, streamlining the synthesis process.
Accurate Predictions: Utilizing the extensive PISTACHIO, BKMS_METABOLIC, PISTACHIO_RINGBREAKER, REAXYS, REAXYS_BIOCATALYSIS database, our tool offers high-accuracy predictions, reflecting the latest in chemical research and data.
Strategy Settings
Precursor scoring | Relevance Heuristic |
---|---|
Min. plausibility | 0.01 |
Model | Template_relevance |
Template Set | Pistachio/Bkms_metabolic/Pistachio_ringbreaker/Reaxys/Reaxys_biocatalysis |
Top-N result to add to graph | 6 |
Feasible Synthetic Routes
Disclaimer and Information on In-Vitro Research Products
Please be aware that all articles and product information presented on BenchChem are intended solely for informational purposes. The products available for purchase on BenchChem are specifically designed for in-vitro studies, which are conducted outside of living organisms. In-vitro studies, derived from the Latin term "in glass," involve experiments performed in controlled laboratory settings using cells or tissues. It is important to note that these products are not categorized as medicines or drugs, and they have not received approval from the FDA for the prevention, treatment, or cure of any medical condition, ailment, or disease. We must emphasize that any form of bodily introduction of these products into humans or animals is strictly prohibited by law. It is essential to adhere to these guidelines to ensure compliance with legal and ethical standards in research and experimentation.