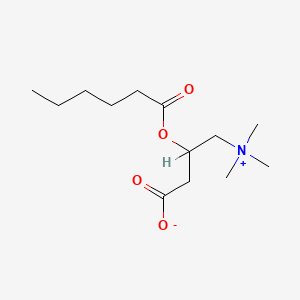
Hexanoylcarnitine
Overview
Description
Hexanoylcarnitine (C6:0) is a medium-chain acylcarnitine with the molecular formula C₁₃H₂₅NO₄ and a molecular weight of 259.346 g/mol . It functions as an intermediate in fatty acid β-oxidation, facilitating the transport of hexanoic acid (a six-carbon fatty acid) into mitochondria for energy production . Elevated levels of this compound are associated with metabolic disorders such as medium-chain acyl-CoA dehydrogenase deficiency (MCADD), sepsis, gastric cancer, and neurodegenerative conditions like Parkinson’s disease . Its structural isomers and derivatives, such as hexenoylcarnitine (C6:1) and 3-hydroxythis compound, further complicate its identification and quantification in metabolomic studies .
Preparation Methods
Synthetic Routes for Hexanoylcarnitine Preparation
Direct Esterification Using Acid Chlorides
The most widely adopted method involves the direct esterification of L-carnitine with hexanoyl chloride. This one-step reaction proceeds via nucleophilic acyl substitution, where the hydroxyl group of carnitine attacks the electrophilic carbonyl carbon of the acid chloride . Key parameters include:
-
Molar Ratios : A slight excess of hexanoyl chloride (1.1–1.3 equivalents) ensures complete conversion of L-carnitine .
-
Solvent Systems : Anhydrous dichloromethane or tetrahydrofuran (THF) is preferred to avoid hydrolysis of the acid chloride .
-
Reaction Duration : Completion typically occurs within 4–6 hours at 25°C, monitored by thin-layer chromatography (TLC) .
For radiolabeled this compound, [³H]carnitine is reacted with hexanoyl chloride in a one-pot synthesis, achieving 90–91% yield after purification .
Carboxylic Acid Activation via Thionyl Chloride
When hexanoyl chloride is unavailable, hexanoic acid is first converted to its acid chloride using thionyl chloride (SOCl₂) :
This step requires refluxing at 70°C for 2 hours under inert gas (e.g., nitrogen) . Subsequent esterification follows the same protocol as Section 1.1.
Reaction Optimization and Kinetic Considerations
Temperature and Catalytic Effects
Elevated temperatures (40–50°C) accelerate the reaction but risk racemization of L-carnitine. Catalysts like 4-dimethylaminopyridine (DMAP) enhance reaction rates by stabilizing the tetrahedral intermediate, reducing reaction times to 2–3 hours .
Solvent Impact on Yield
Polar aprotic solvents (e.g., acetonitrile) improve reagent solubility but may necessitate longer purification steps. Nonpolar solvents (e.g., heptane) facilitate phase separation during workup, favoring industrial scalability .
Table 1: Solvent Comparison for this compound Synthesis
Solvent | Reaction Time (h) | Yield (%) | Purity (%) |
---|---|---|---|
Dichloromethane | 4.0 | 88 | 95 |
THF | 3.5 | 85 | 93 |
Acetonitrile | 2.5 | 90 | 97 |
Purification and Isolation Techniques
Liquid-Liquid Partitioning
Crude reaction mixtures are treated with isopropanol:hydrochloric acid:heptane (4:1:12 v/v) to separate this compound from unreacted carnitine and acid chloride byproducts . This step achieves >90% recovery with <1% carnitine contamination .
Anion Exchange Chromatography
To obtain the inner salt form, the product is passed through a weakly basic anion exchanger (e.g., Dowex 1×8-200), replacing chloride counterions with hydroxide . This step is critical for applications requiring neutral pH compatibility.
Column Chromatography
Silica gel chromatography with eluents like chloroform:methanol:ammonia (75:25:4 v/v) removes trace impurities, yielding >98% pure this compound .
Industrial-Scale Production Considerations
Continuous Flow Synthesis
Automated reactors with in-line monitoring (e.g., FTIR spectroscopy) enable real-time adjustment of stoichiometry and temperature, ensuring batch-to-batch consistency .
Cost-Efficiency Analysis
Component | Laboratory Cost (USD/g) | Industrial Cost (USD/g) |
---|---|---|
L-Carnitine | 12.50 | 8.20 |
Hexanoyl Chloride | 9.80 | 6.50 |
Solvents | 3.20 | 1.10 |
Economies of scale reduce production costs by 35–40% in industrial settings .
Analytical Validation of Synthesized Product
Thin-Layer Chromatography (TLC)
Rf values of 0.45–0.50 (silica gel, chloroform:methanol:water 65:25:4) confirm product identity .
Liquid Chromatography-Mass Spectrometry (LC-MS)
-
Column : C18, 2.1 × 100 mm, 1.7 µm
-
Mobile Phase : 0.1% formic acid in acetonitrile/water (70:30)
-
Detection : m/z 259.18 [M+H]⁺, characteristic fragments at m/z 200.12 (loss of CO₂) and m/z 144.08 (carnitine backbone) .
Challenges and Methodological Limitations
Racemization Risks
Prolonged heating above 50°C induces racemization, reducing biological activity. Strict temperature control (<40°C) is essential .
Hydrolytic Instability
This compound undergoes hydrolysis at pH <2 or >9, necessitating neutral storage conditions (pH 6.5–7.5) .
Scalability of Radiolabeled Synthesis
High specific activity [³H]this compound requires specialized facilities for handling tritium, increasing production costs by 20–25% .
Chemical Reactions Analysis
Types of Reactions: Hexanoylcarnitine undergoes various chemical reactions, including:
Oxidation: It can be oxidized to form corresponding carboxylic acids.
Reduction: Reduction reactions can convert it back to carnitine and hexanoic acid.
Substitution: Nucleophilic substitution reactions can replace the ester group with other functional groups.
Common Reagents and Conditions:
Oxidation: Common oxidizing agents include potassium permanganate (KMnO₄) and chromium trioxide (CrO₃).
Reduction: Reducing agents like lithium aluminum hydride (LiAlH₄) are used.
Substitution: Nucleophiles such as hydroxide ions (OH⁻) or amines are employed.
Major Products:
Oxidation: Hexanoic acid and carnitine.
Reduction: Carnitine and hexanoic acid.
Substitution: Various substituted carnitine derivatives.
Scientific Research Applications
Medical Science
1.1 Diabetic Cardiomyopathy Research
Hexanoylcarnitine is being investigated as a biomarker for diabetic cardiomyopathy (DCM). A study involving plasma samples from patients with type 2 diabetes and DCM revealed that elevated levels of this compound correlate positively with the risk of DCM. This suggests its potential utility in early diagnosis and monitoring of the disease.
1.2 Metabolic Disorders
This compound plays a crucial role in diagnosing various metabolic disorders. Its levels are often elevated in conditions such as medium-chain acyl-CoA dehydrogenase deficiency, which highlights its diagnostic relevance. The measurement of acylcarnitines, including this compound, can provide insights into energy production disturbances and intermediary metabolism in patients .
1.3 Kidney Disease
In nephrology, this compound is essential for fatty acid metabolism and mitochondrial function. It is particularly relevant for patients with kidney disease, where deficiencies arise due to reduced renal synthesis and dietary restrictions. Supplementation with L-carnitine, including this compound, has been suggested to mitigate these deficiencies and improve metabolic functions.
Sports Science and Nutrition
2.1 Athletic Performance Enhancement
This compound is utilized as a dietary supplement among athletes to enhance performance through improved fatty acid metabolism. Research indicates that prolonged supplementation can increase total carnitine content in skeletal muscle, potentially leading to enhanced endurance and recovery during intense training sessions.
2.2 Metabolomics in Nutrition
Recent metabolomic studies have demonstrated that this compound levels increase significantly following low-calorie diets, correlating with reductions in visceral fat area. This underscores its role as a biomarker for dietary interventions aimed at weight loss and metabolic health improvement .
Table 1: Summary of Research Findings on this compound
Mechanism of Action
Hexanoylcarnitine facilitates the transport of fatty acids into the mitochondria, where they undergo β-oxidation to produce energy. It acts by forming a complex with fatty acids, which is then transported across the mitochondrial membrane by carnitine-acylcarnitine translocase. Inside the mitochondria, the fatty acids are released and undergo β-oxidation, generating acetyl-CoA, which enters the citric acid cycle to produce ATP .
Comparison with Similar Compounds
Structural and Functional Differences
Acylcarnitines vary by chain length, saturation, and functional groups, which dictate their metabolic roles and clinical significance. Below is a comparative analysis of hexanoylcarnitine with key analogs:
Data Table 1: Comparative Analysis of Acylcarnitines
Clinical and Metabolic Contexts
- MCADD: this compound (C6:0), octanoylcarnitine (C8:0), and decanoylcarnitine (C10:0) are elevated in MCADD due to defective fatty acid oxidation. C8:0 is the primary biomarker, but C6:0 and C10:0 provide supplementary diagnostic insights .
- Sepsis Prognosis: this compound levels (20.3 mg/dL in nonsurvivors vs. 24.6 mg/dL in controls) are part of a panel with butyroylcarnitine (C4:0) and 4-cis-decenoylcarnitine (C10:1), where shorter-chain species (C4:0) show stronger associations with mortality .
- Cancer Biomarkers: In gastric cancer, this compound (C6:0) is elevated alongside adipylcarnitine (C6DC; AUC = 0.9977 for diagnosis).
- Neurodegeneration: this compound accumulation in Parkinson’s disease models correlates with oxidative stress and glutathione depletion, whereas acetyl-threonine (another metabolite) links to neuronal growth .
Analytical Challenges
This compound has five structural isomers (e.g., C6:0-a, C6:0-b), complicating its identification in untargeted metabolomics . In contrast, butyroylcarnitine (C4:0) and propionylcarnitine (C3:0) exhibit fewer isomers, enabling clearer quantification .
Dietary and Pharmacological Influences
- Ketogenic Diets: this compound levels decrease during ketogenic interventions, reflecting increased fatty acid utilization, unlike stearoylcarnitine (C18:0), which remains stable .
Biological Activity
Hexanoylcarnitine, a medium-chain acylcarnitine, plays significant roles in cellular metabolism, particularly in fatty acid transport and energy production. This article explores its biological activity, mechanisms of action, and implications for health, supported by research findings and data tables.
Overview of this compound
This compound is formed from the esterification of carnitine with hexanoic acid. It is primarily involved in the transport of fatty acids into mitochondria for β-oxidation, a critical process for energy production. Its biological functions extend beyond mere transport; it is implicated in various metabolic pathways and physiological processes.
- Mitochondrial Fatty Acid Transport : this compound inhibits mitochondrial fatty acid transport by interfering with the carnitine palmitoyltransferase (CPT) system. Research indicates that it specifically affects the carnitine-acylcarnitine translocase (CACT), which is crucial for the transport of acylcarnitines across mitochondrial membranes. The inhibitory potency of this compound is relatively low compared to other acylcarnitines, with an IC50 greater than 200 µM, suggesting a less pronounced effect on fatty acid oxidation compared to longer-chain acylcarnitines like decanoylcarnitine .
- Influence on Metabolic Profiles : Studies have shown that this compound levels can increase under certain dietary conditions, such as caloric restriction or low-calorie diets. For example, a 12-week low-calorie diet resulted in significant increases in plasma levels of this compound alongside other medium- and long-chain acylcarnitines, correlating with reductions in visceral fat area .
- Role in Lipid Metabolism : this compound is involved in lipid metabolism regulation. In conditions like familial combined hyperlipidemia, elevated levels of this compound have been associated with altered lipid profiles and may serve as potential biomarkers for metabolic disturbances .
Case Study 1: Caloric Restriction and Acylcarnitine Profiles
A study examining the effects of caloric restriction on liver metabolism found that this compound levels increased significantly alongside other acylcarnitines. This shift suggests that caloric restriction enhances fatty acid oxidation pathways and alters acylcarnitine profiles to promote metabolic flexibility .
Case Study 2: Metabolomic Profiling in Disease States
Research utilizing multiplatform plasma metabolic profiling identified increases in this compound among other metabolites in patients with breast cancer. This finding highlights the potential role of this compound as a metabolic marker in disease states, indicating its involvement in altered lipid metabolism associated with cancer .
Data Table: Biological Activity Summary
Biological Activity | Mechanism | IC50 (µM) | Clinical Relevance |
---|---|---|---|
Mitochondrial Fatty Acid Transport | Inhibits CACT activity | >200 | Implicated in energy metabolism disorders |
Lipid Metabolism Regulation | Alters acylcarnitine profiles under caloric restriction | N/A | Potential biomarker for metabolic disorders |
Association with Disease | Elevated levels noted in breast cancer studies | N/A | Possible role in cancer metabolism |
Q & A
Q. What are the primary methodological approaches for detecting and quantifying hexanoylcarnitine in biological samples?
Basic Research Focus
this compound is predominantly analyzed using liquid chromatography-mass spectrometry (LC-MS) due to its high sensitivity and specificity for acylcarnitines. Key steps include:
- Sample Preparation : Deproteinization of serum/plasma via organic solvents (e.g., methanol or acetonitrile) to isolate metabolites .
- LC-MS Parameters : Reverse-phase chromatography (C18 columns) with electrospray ionization (ESI) in positive ion mode. This compound is identified by its m/z 259.18 [M+H]⁺ ion and characteristic fragmentation patterns (e.g., loss of 59 Da for carnitine backbone cleavage) .
- Validation : Use of deuterated internal standards (e.g., d₃-hexanoylcarnitine) to correct for matrix effects and ensure reproducibility .
Q. How is this compound implicated in mitochondrial dysfunction, and what experimental evidence supports this role?
Basic Research Focus
this compound accumulates during impaired β-oxidation, reflecting mitochondrial dysfunction. Key evidence includes:
- In Vivo Studies : Elevated serum this compound in acute-on-chronic liver failure patients correlates with mitochondrial damage, validated via LC-MS and clinical outcomes (e.g., mortality risk) .
- Genetic Disorders : MCADD (Medium-Chain Acyl-CoA Dehydrogenase Deficiency) patients show 10–20× higher this compound levels in neonatal screenings, confirmed by tandem MS and ACADM gene mutation analysis .
- Mechanistic Insights : In vitro models using hepatocytes treated with mitochondrial toxins (e.g., rotenone) replicate this compound accumulation, linking it to ATP depletion and ROS overproduction .
Q. What experimental design considerations are critical when validating this compound as a biomarker in metabolic disorders?
Advanced Research Focus
Validation requires rigorous cohort selection and analytical validation:
- Cohort Design :
- Analytical Rigor :
Q. How can researchers resolve contradictions in this compound levels observed across different clinical studies?
Advanced Research Focus
Discrepancies (e.g., elevated vs. reduced levels) arise from contextual factors:
- Disease Heterogeneity : In sepsis, this compound may decrease due to systemic carnitine depletion, while liver failure shows increases from mitochondrial stress .
- Methodological Variability : Differences in sample handling (e.g., fasting vs. non-fasting) or LC-MS ionization efficiency can skew results. Standardized pre-analytical protocols are critical .
- Multi-Omics Integration : Pair metabolomics with transcriptomic data (e.g., CPT1A expression) to contextualize this compound fluctuations within metabolic pathways .
Q. What computational and statistical methods are employed to integrate this compound data into multi-omics prognostic models?
Advanced Research Focus
this compound is incorporated into predictive models using:
- Machine Learning : LASSO regression selects this compound as a top biomarker in mortality risk models (e.g., CLIF-C MET model for liver failure: log₂(this compound) coefficient = 0.456) .
- Pathway Enrichment : Metabolite Set Enrichment Analysis (MSEA) links this compound to urea cycle dysregulation and immune-metabolic crosstalk in sepsis .
- Network Analysis : Gaussian graphical models reveal correlations between this compound and inflammatory markers (e.g., IL-6) in T2DM cohorts .
Q. What are the key steps in synthesizing and characterizing this compound derivatives for functional studies?
Advanced Research Focus
Synthesis and characterization involve:
- Chemical Synthesis :
- Functional Assays :
Properties
IUPAC Name |
3-hexanoyloxy-4-(trimethylazaniumyl)butanoate | |
---|---|---|
Source | PubChem | |
URL | https://pubchem.ncbi.nlm.nih.gov | |
Description | Data deposited in or computed by PubChem | |
InChI |
InChI=1S/C13H25NO4/c1-5-6-7-8-13(17)18-11(9-12(15)16)10-14(2,3)4/h11H,5-10H2,1-4H3 | |
Source | PubChem | |
URL | https://pubchem.ncbi.nlm.nih.gov | |
Description | Data deposited in or computed by PubChem | |
InChI Key |
VVPRQWTYSNDTEA-UHFFFAOYSA-N | |
Source | PubChem | |
URL | https://pubchem.ncbi.nlm.nih.gov | |
Description | Data deposited in or computed by PubChem | |
Canonical SMILES |
CCCCCC(=O)OC(CC(=O)[O-])C[N+](C)(C)C | |
Source | PubChem | |
URL | https://pubchem.ncbi.nlm.nih.gov | |
Description | Data deposited in or computed by PubChem | |
Molecular Formula |
C13H25NO4 | |
Source | PubChem | |
URL | https://pubchem.ncbi.nlm.nih.gov | |
Description | Data deposited in or computed by PubChem | |
DSSTOX Substance ID |
DTXSID50982706 | |
Record name | 3-(Hexanoyloxy)-4-(trimethylazaniumyl)butanoate | |
Source | EPA DSSTox | |
URL | https://comptox.epa.gov/dashboard/DTXSID50982706 | |
Description | DSSTox provides a high quality public chemistry resource for supporting improved predictive toxicology. | |
Molecular Weight |
259.34 g/mol | |
Source | PubChem | |
URL | https://pubchem.ncbi.nlm.nih.gov | |
Description | Data deposited in or computed by PubChem | |
CAS No. |
6418-78-6 | |
Record name | Hexanoylcarnitine | |
Source | CAS Common Chemistry | |
URL | https://commonchemistry.cas.org/detail?cas_rn=6418-78-6 | |
Description | CAS Common Chemistry is an open community resource for accessing chemical information. Nearly 500,000 chemical substances from CAS REGISTRY cover areas of community interest, including common and frequently regulated chemicals, and those relevant to high school and undergraduate chemistry classes. This chemical information, curated by our expert scientists, is provided in alignment with our mission as a division of the American Chemical Society. | |
Explanation | The data from CAS Common Chemistry is provided under a CC-BY-NC 4.0 license, unless otherwise stated. | |
Record name | 3-(Hexanoyloxy)-4-(trimethylazaniumyl)butanoate | |
Source | EPA DSSTox | |
URL | https://comptox.epa.gov/dashboard/DTXSID50982706 | |
Description | DSSTox provides a high quality public chemistry resource for supporting improved predictive toxicology. | |
Retrosynthesis Analysis
AI-Powered Synthesis Planning: Our tool employs the Template_relevance Pistachio, Template_relevance Bkms_metabolic, Template_relevance Pistachio_ringbreaker, Template_relevance Reaxys, Template_relevance Reaxys_biocatalysis model, leveraging a vast database of chemical reactions to predict feasible synthetic routes.
One-Step Synthesis Focus: Specifically designed for one-step synthesis, it provides concise and direct routes for your target compounds, streamlining the synthesis process.
Accurate Predictions: Utilizing the extensive PISTACHIO, BKMS_METABOLIC, PISTACHIO_RINGBREAKER, REAXYS, REAXYS_BIOCATALYSIS database, our tool offers high-accuracy predictions, reflecting the latest in chemical research and data.
Strategy Settings
Precursor scoring | Relevance Heuristic |
---|---|
Min. plausibility | 0.01 |
Model | Template_relevance |
Template Set | Pistachio/Bkms_metabolic/Pistachio_ringbreaker/Reaxys/Reaxys_biocatalysis |
Top-N result to add to graph | 6 |
Feasible Synthetic Routes
Disclaimer and Information on In-Vitro Research Products
Please be aware that all articles and product information presented on BenchChem are intended solely for informational purposes. The products available for purchase on BenchChem are specifically designed for in-vitro studies, which are conducted outside of living organisms. In-vitro studies, derived from the Latin term "in glass," involve experiments performed in controlled laboratory settings using cells or tissues. It is important to note that these products are not categorized as medicines or drugs, and they have not received approval from the FDA for the prevention, treatment, or cure of any medical condition, ailment, or disease. We must emphasize that any form of bodily introduction of these products into humans or animals is strictly prohibited by law. It is essential to adhere to these guidelines to ensure compliance with legal and ethical standards in research and experimentation.