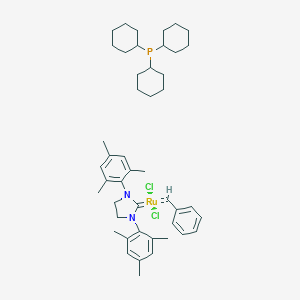
Grubbs Catalyst 2nd Generation
Overview
Description
Grubbs Catalyst 2nd Generation (C₂) is a ruthenium-based olefin metathesis catalyst with the formula C₄₆H₆₅Cl₂N₂PRu (CAS: 246047-72-3) . It is a modified version of the 1st generation catalyst, where the labile tricyclohexylphosphine (PCy₃) ligand is replaced by a stronger σ-donor, 1,3-bis(2,4,6-trimethylphenyl)imidazolinium (H₂IMes), an N-heterocyclic carbene (NHC) ligand (Fig. 1) . This substitution enhances thermal stability, catalytic activity, and functional group tolerance, making it versatile for applications in:
- Ring-closing metathesis (RCM)
- Cross-metathesis (CM)
- Ring-opening metathesis polymerization (ROMP)
- Acyclic diene metathesis (ADMET) .
Key structural features include a ruthenium center coordinated to two chloride ligands, a benzylidene group, and the NHC ligand. The electronic properties of the Ru center are influenced by ligand interactions, such as hydrogen bonding or coordination with derivatives like p-cresol .
Preparation Methods
The synthesis of Grubbs Catalyst 2nd Generation involves the reaction of a first-generation Grubbs catalyst with a specific ligand. The process typically includes the following steps:
Starting Material: The first-generation Grubbs catalyst, which is a ruthenium complex.
Ligand Addition: A ligand such as 1,3-bis(2,4,6-trimethylphenyl)-2-imidazolidinylidene is added to the reaction mixture.
Reaction Conditions: The reaction is carried out under an inert atmosphere, often using nitrogen or argon, at a controlled temperature.
Purification: The resulting product is purified using techniques such as crystallization or chromatography.
Industrial production methods for this compound are similar but are scaled up to accommodate larger quantities. These methods often involve continuous flow processes and automated systems to ensure consistency and efficiency .
Chemical Reactions Analysis
Catalytic Mechanism and Active Species Formation
The second-generation Grubbs catalyst initiates metathesis through phosphine ligand dissociation, forming a 14-electron active species. This process is slower than in first-generation catalysts but is offset by stronger substrate affinity due to the NHC ligand’s σ-donor and π-acceptor properties . The NHC ligand increases the lability of the remaining tricyclohexylphosphine (PCy₃) ligand, facilitating faster entry into the catalytic cycle .
The active 14-electron species coordinates olefins to form a ruthenacyclobutane intermediate (Figure 1), which undergoes [2+2] cycloaddition and cycloreversion steps. Density functional theory (DFT) studies reveal that cycloaddition with GII has activation free energies (ΔG‡) as low as 2.4 kcal/mol , significantly lower than first-generation catalysts (ΔG‡ = 8.5 kcal/mol) .
Reaction Energetics and Stability
Comparative studies using the Unified Reaction Valley Approach (URVA) highlight the thermodynamic and kinetic advantages of GII:
Reaction Step | Catalyst Model | ΔG‡ (kcal/mol) | ΔGᵣ (kcal/mol) |
---|---|---|---|
[2+2] Cycloaddition | M1 (GI model) | 8.5 | 0.6 |
M2 (GII model) | 6.0 | -1.3 | |
M3 (GII+steric) | 2.4 | -12.2 | |
Cycloreversion | M1 | 11.7 | -1.7 |
M2 | 12.8 | 1.1 | |
M3 | 17.1 | 8.3 |
Table 1: Energetics of cycloaddition and cycloreversion steps for Grubbs catalysts .
Spectroscopic and Electrochemical Behavior
UV-vis spectroscopy (0.10 mM in CH₂Cl₂) reveals a faster transformation rate for GII (kₒbₛ = 1.52 × 10⁻⁴ s⁻¹) compared to first-generation catalysts (kₒbₛ = 7.48 × 10⁻⁵ s⁻¹) . Despite this, cyclic voltammetry shows GII’s Ru(II)/Ru(III) oxidation potential (E°’ = 27.5 mV) is more positive than first-generation catalysts, indicating greater resistance to oxidation .
Comparative Performance with First-Generation Catalysts
Property | Grubbs First-Gen | Grubbs Second-Gen |
---|---|---|
Ligands | 2 PCy₃ | 1 PCy₃, 1 NHC |
Thermal Stability | Moderate | High |
Cycloaddition ΔG‡ | 8.5 kcal/mol | 2.4 kcal/mol |
Substrate Tolerance | Limited | Broad |
Table 2: Key differences between first- and second-generation Grubbs catalysts .
Scientific Research Applications
Applications in Scientific Research
Grubbs Catalyst GII plays a crucial role in the synthesis of complex pharmaceutical compounds through various metathesis reactions, including cross-metathesis and ring-closing metathesis.
Case Study: Synthesis of Anticancer Agents
In a study published in ACS Omega, researchers utilized GII to synthesize key intermediates for anticancer agents. The catalyst facilitated the formation of cyclic compounds with high yields and selectivity.
- Target Compound : A novel anticancer agent
- Metathesis Reaction : Ring-closing metathesis
- Yield : 92%
- Reaction Time : 4 hours
Materials Science
GII is also employed in developing advanced materials, including nanocomposites and functionalized polymers. Its ability to create complex structures makes it invaluable in creating materials with specific properties.
Application Example: Nanocomposites
Researchers have demonstrated that GII can be used to synthesize nanocomposites by incorporating nanoparticles into polymer matrices through metathesis reactions.
Material Type | Composition | Properties |
---|---|---|
Conductive Polymer | Polyaniline + Nanoparticles | Enhanced electrical conductivity |
Biodegradable Plastic | PLA + Bioceramic Nanoparticles | Improved mechanical strength |
Mechanism of Action
The mechanism of action of Grubbs Catalyst 2nd Generation involves the formation of a metallacyclobutane intermediate. The process begins with the coordination of the olefin to the ruthenium center, followed by the formation of a metallacyclobutane ring. This intermediate then breaks down to form the desired product and regenerate the catalyst. The molecular targets and pathways involved include the carbon-carbon double bonds of the olefins and the ruthenium center of the catalyst .
Comparison with Similar Compounds
Comparative Analysis with Similar Catalysts
Grubbs 1st Generation (C₁)
Key Insight : C₂’s NHC ligand enhances σ-donation, stabilizing the Ru center and accelerating metathesis rates. C₁ is less suitable for sterically demanding or electron-deficient olefins.
Hoveyda-Grubbs 2nd Generation (HG₂)
Key Insight : HG₂’s chelating ether ligand improves thermal stability and recyclability but sacrifices activity and selectivity in cross-metathesis.
Schrock Catalysts (Mo/W-based)
Key Insight : Schrock catalysts outperform C₂ in stereochemical control but require stringent handling, limiting industrial use.
Modified Grubbs Catalysts
- Pyridine-Modified C₂ : Enables copolymerization reactions inaccessible to standard C₂ .
- p-Cresol Derivatives : Alter electronic properties via Ru-O coordination or hydrogen bonding, tuning reactivity for specific substrates .
Critical Research Findings
Catalytic Efficiency
- In cross-metathesis of methyl vinyl glycolate (MVG), C₂ achieved 62% yield vs. 25% for C₁ and 44% for HG₂ .
- C₂ outperforms HG₂ in ethylene-based ROMP, producing higher molecular weight polymers with minimal isomerization .
Decomposition Pathways
- C₂ resists β-hydride elimination (ΔG‡ = 24.3 kcal/mol) better than C₁ (ΔG‡ = 16.9 kcal/mol), reducing deactivation .
Ligand Effects
- NHC ligands in C₂ increase electron density at Ru, enhancing turnover frequency (TOF) in RCM .
- p-Cresol derivatives modify chloride ligand interactions, enabling tailored catalyst design for niche applications .
Biological Activity
The Grubbs Catalyst 2nd Generation , also known as the Hoveyda-Grubbs catalyst, is a highly efficient organometallic compound primarily used in olefin metathesis reactions. Its structure includes a ruthenium center coordinated to a saturated N-heterocyclic carbene (NHC) and phosphine ligands, which enhances its reactivity compared to first-generation catalysts. This article explores the biological activity of this catalyst, focusing on its applications, mechanisms, and potential biological effects.
Structure and Mechanism
The second-generation Grubbs catalyst is represented by the formula and has a molecular weight of approximately 848.98 g/mol . The catalyst operates through a mechanism involving the reversible dissociation of phosphine ligands, leading to an active catalytic complex that can coordinate olefins to form ruthenacyclobutane intermediates . This mechanism allows for efficient ring-closing and cross-metathesis reactions.
Biological Activity
Recent studies have indicated that the Grubbs catalyst may exhibit biological activity beyond its synthetic applications. Notably, research has explored its potential to trigger biological effects, particularly in the context of drug development and biocatalysis.
Case Studies
-
Catalyst Conjugation with Enzymes :
- A study investigated the conjugation of the Hoveyda-Grubbs catalyst with lipase from Candida antarctica (CAL-B). The resulting hybrid catalyst demonstrated significant activity in ring-closing metathesis reactions even in aqueous media, achieving up to 50% conversion within 2 hours . This suggests that the biological environment can influence the catalytic efficiency and stability of organometallic complexes.
-
Heterogeneous Catalysis :
- The immobilization of the Grubbs catalyst on functionalized supports has been shown to maintain catalytic activity while allowing for easier separation and reuse. For instance, a boronic acid-functionalized support facilitated 50% conversion after 96 hours in a ring-closing metathesis reaction . This approach highlights the potential for biocompatibility and environmental applications.
-
Toxicity and Safety :
- Investigations into the safety profile of the Grubbs catalyst indicate that while it is effective in synthetic applications, there are concerns regarding its toxicity and environmental impact. Studies have raised awareness about the need for careful handling due to potential hazards associated with ruthenium complexes .
Comparative Data
The following table summarizes key characteristics and findings related to the Grubbs catalyst's biological activity:
Parameter | This compound | First Generation Catalyst |
---|---|---|
Molecular Formula | C46H65Cl2N2PRu | C40H52Cl2N2PRu |
Molecular Weight | 848.98 g/mol | 774.47 g/mol |
Activity in Ring-Closing Metathesis | High (up to 50% conversion in 2h) | Moderate |
Biological Applications | Enzyme conjugation, biocatalysis | Limited |
Toxicity Concerns | Present | Present |
Research Findings
- Catalytic Efficiency : The second-generation Grubbs catalyst exhibits significantly higher activity in various metathesis reactions compared to its predecessor, making it suitable for complex organic syntheses .
- Mechanistic Insights : Studies have shown that this catalyst initiates metathesis reactions more slowly but achieves greater efficiency due to its higher affinity for olefinic substrates .
- Environmental Impact : There is ongoing research into reducing the environmental footprint of using such catalysts by exploring their recyclability and stability under various conditions .
Q & A
Basic Research Questions
Q. What distinguishes Grubbs Catalyst 2nd Generation from the 1st Generation in terms of structure and activity?
The 2nd Generation catalyst replaces one tricyclohexylphosphine (PCy3) ligand in the 1st Generation with a saturated N-heterocyclic carbene (NHC) ligand. This NHC ligand provides stronger σ-donation, enhancing catalytic activity and substrate scope, particularly for sterically hindered or electron-deficient alkenes . The improved activity allows for lower catalyst loadings in reactions like cross-metathesis (CM) and ring-closing metathesis (RCM) .
Q. What safety precautions are critical when handling this compound?
The catalyst is air-sensitive and must be stored and handled under inert atmospheres (e.g., nitrogen or argon). Protective equipment—gloves, lab coats, and eye protection—is mandatory due to its flammability (H228 hazard code). Waste must be segregated and disposed of via specialized chemical waste services to avoid environmental contamination .
Q. Which reaction types are most effectively catalyzed by this compound?
It is widely used in:
- Ring-closing metathesis (RCM): Synthesis of medium-to-large cyclic compounds (e.g., macrocycles).
- Cross-metathesis (CM): Coupling of terminal or internal alkenes.
- Ring-opening metathesis polymerization (ROMP): Production of functionalized polymers . Its tolerance for polar functional groups (e.g., esters, amides) enables applications in complex molecule synthesis .
Q. How should reaction solvents be selected for optimal metathesis performance?
Dichloromethane (DCM) and toluene are common due to their inertness and ability to dissolve the catalyst. Protic solvents (e.g., methanol) are generally avoided, as they can deactivate the catalyst. Solvent choice should align with substrate solubility and reaction temperature requirements .
Advanced Research Questions
Q. What experimental strategies mitigate catalyst decomposition during prolonged reactions?
Decomposition pathways include ligand dissociation and oxidative degradation. Strategies include:
- Low-temperature initiation: Reduces thermal degradation (e.g., 40–60°C for CM).
- Inert atmosphere maintenance: Prevents oxidation of the Ru center.
- Additives: Lewis acids (e.g., Ti(OiPr)4) stabilize the active Ru-carbene intermediate . Monitoring via <sup>31</sup>P NMR can track phosphine ligand dissociation, a sign of decomposition .
Q. How do electronic and steric effects of substrates influence metathesis efficiency?
- Electron-deficient alkenes (e.g., α,β-unsaturated carbonyls) require the 2nd Generation catalyst’s enhanced electron-donating NHC ligand to stabilize transition states.
- Sterically hindered substrates (e.g., 1,1-disubstituted alkenes) benefit from the NHC ligand’s bulk tolerance, which reduces steric clash compared to the 1st Generation . Kinetic studies using <sup>1</sup>H NMR or GC-MS can quantify reaction rates under varying steric/electronic conditions .
Q. What analytical methods are recommended for characterizing metathesis products and catalyst integrity?
- <sup>1</sup>H NMR: Tracks alkene proton shifts to confirm metathesis progression.
- GC-MS/HPLC: Identifies side products (e.g., homodimers in CM).
- X-ray crystallography: Resolves catalyst-substrate adduct structures.
- UV-Vis spectroscopy: Monitors Ru-carbene intermediates in real time .
Q. How can substrate-catalyst interactions be optimized for challenging RCM reactions?
- Substrate preorganization: Introducing directing groups (e.g., hydrogen-bond acceptors) aligns alkenes for cyclization.
- Microwave-assisted synthesis: Accelerates slow RCM steps, reducing decomposition.
- Computational modeling: DFT calculations predict favorable transition states and guide substrate design .
Q. What are the limitations of this compound in stereoselective metathesis?
While effective for E/Z control in simple alkenes, stereoselectivity diminishes with highly substituted substrates. Chiral NHC ligands or Hoveyda-Grubbs variants (with chelating ether groups) improve enantioselectivity in asymmetric metathesis .
Properties
IUPAC Name |
benzylidene-[1,3-bis(2,4,6-trimethylphenyl)imidazolidin-2-ylidene]-dichlororuthenium;tricyclohexylphosphane | |
---|---|---|
Source | PubChem | |
URL | https://pubchem.ncbi.nlm.nih.gov | |
Description | Data deposited in or computed by PubChem | |
InChI |
InChI=1S/C21H26N2.C18H33P.C7H6.2ClH.Ru/c1-14-9-16(3)20(17(4)10-14)22-7-8-23(13-22)21-18(5)11-15(2)12-19(21)6;1-4-10-16(11-5-1)19(17-12-6-2-7-13-17)18-14-8-3-9-15-18;1-7-5-3-2-4-6-7;;;/h9-12H,7-8H2,1-6H3;16-18H,1-15H2;1-6H;2*1H;/q;;;;;+2/p-2 | |
Source | PubChem | |
URL | https://pubchem.ncbi.nlm.nih.gov | |
Description | Data deposited in or computed by PubChem | |
InChI Key |
FCDPQMAOJARMTG-UHFFFAOYSA-L | |
Source | PubChem | |
URL | https://pubchem.ncbi.nlm.nih.gov | |
Description | Data deposited in or computed by PubChem | |
Canonical SMILES |
CC1=CC(=C(C(=C1)C)N2CCN(C2=[Ru](=CC3=CC=CC=C3)(Cl)Cl)C4=C(C=C(C=C4C)C)C)C.C1CCC(CC1)P(C2CCCCC2)C3CCCCC3 | |
Source | PubChem | |
URL | https://pubchem.ncbi.nlm.nih.gov | |
Description | Data deposited in or computed by PubChem | |
Molecular Formula |
C46H65Cl2N2PRu | |
Source | PubChem | |
URL | https://pubchem.ncbi.nlm.nih.gov | |
Description | Data deposited in or computed by PubChem | |
Molecular Weight |
849.0 g/mol | |
Source | PubChem | |
URL | https://pubchem.ncbi.nlm.nih.gov | |
Description | Data deposited in or computed by PubChem | |
CAS No. |
246047-72-3 | |
Record name | Ruthenium, [1,3-bis(2,4,6-trimethylphenyl)-2-imidazolidinylidene]dichloro(phenylmethylene)(tricyclohexylphosphine)-, (SP-5-41)- | |
Source | EPA Chemicals under the TSCA | |
URL | https://www.epa.gov/chemicals-under-tsca | |
Description | EPA Chemicals under the Toxic Substances Control Act (TSCA) collection contains information on chemicals and their regulations under TSCA, including non-confidential content from the TSCA Chemical Substance Inventory and Chemical Data Reporting. | |
Record name | (1,3-Bis(2,4,6-trimethylphenyl)-2-imidazolidinylidene)dichloro(phenylmethylene)(tricyclohexylphosphine)ruthenium Benzylidene[1,3-bis(2,4,6-trimethylphenyl)-2-imidazolidinylidene]dichloro(tricyclohexylphosphine)ruthenium [1,3-Bis(2,4,6-trimethylphenyl)-2-imidazolidinylidene]diidazolidinylidene)(dichlorophenylmethylene)(tricyclohexylphosphine)ruthenium | |
Source | European Chemicals Agency (ECHA) | |
URL | https://echa.europa.eu/information-on-chemicals | |
Description | The European Chemicals Agency (ECHA) is an agency of the European Union which is the driving force among regulatory authorities in implementing the EU's groundbreaking chemicals legislation for the benefit of human health and the environment as well as for innovation and competitiveness. | |
Explanation | Use of the information, documents and data from the ECHA website is subject to the terms and conditions of this Legal Notice, and subject to other binding limitations provided for under applicable law, the information, documents and data made available on the ECHA website may be reproduced, distributed and/or used, totally or in part, for non-commercial purposes provided that ECHA is acknowledged as the source: "Source: European Chemicals Agency, http://echa.europa.eu/". Such acknowledgement must be included in each copy of the material. ECHA permits and encourages organisations and individuals to create links to the ECHA website under the following cumulative conditions: Links can only be made to webpages that provide a link to the Legal Notice page. | |
Retrosynthesis Analysis
AI-Powered Synthesis Planning: Our tool employs the Template_relevance Pistachio, Template_relevance Bkms_metabolic, Template_relevance Pistachio_ringbreaker, Template_relevance Reaxys, Template_relevance Reaxys_biocatalysis model, leveraging a vast database of chemical reactions to predict feasible synthetic routes.
One-Step Synthesis Focus: Specifically designed for one-step synthesis, it provides concise and direct routes for your target compounds, streamlining the synthesis process.
Accurate Predictions: Utilizing the extensive PISTACHIO, BKMS_METABOLIC, PISTACHIO_RINGBREAKER, REAXYS, REAXYS_BIOCATALYSIS database, our tool offers high-accuracy predictions, reflecting the latest in chemical research and data.
Strategy Settings
Precursor scoring | Relevance Heuristic |
---|---|
Min. plausibility | 0.01 |
Model | Template_relevance |
Template Set | Pistachio/Bkms_metabolic/Pistachio_ringbreaker/Reaxys/Reaxys_biocatalysis |
Top-N result to add to graph | 6 |
Feasible Synthetic Routes
Disclaimer and Information on In-Vitro Research Products
Please be aware that all articles and product information presented on BenchChem are intended solely for informational purposes. The products available for purchase on BenchChem are specifically designed for in-vitro studies, which are conducted outside of living organisms. In-vitro studies, derived from the Latin term "in glass," involve experiments performed in controlled laboratory settings using cells or tissues. It is important to note that these products are not categorized as medicines or drugs, and they have not received approval from the FDA for the prevention, treatment, or cure of any medical condition, ailment, or disease. We must emphasize that any form of bodily introduction of these products into humans or animals is strictly prohibited by law. It is essential to adhere to these guidelines to ensure compliance with legal and ethical standards in research and experimentation.