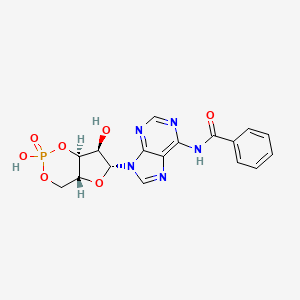
Bz-Camp
Overview
Description
Bz-cAMP (Benzoyl cyclic adenosine monophosphate) is a synthetic cyclic AMP (cAMP) analog widely used in biochemical studies to investigate cAMP-dependent signaling pathways, particularly those mediated by protein kinase A (PKA). Structurally, this compound retains the core cAMP scaffold but incorporates a benzoyl group at the N6 position of the adenine ring (Figure 1). This modification enhances its stability against phosphodiesterase degradation and increases membrane permeability compared to unmodified cAMP .
This compound acts as a potent activator of PKA by binding to the regulatory (R) subunits, inducing conformational changes that release the catalytic (C) subunits. This activation facilitates downstream phosphorylation events critical for cellular processes such as metabolism, gene expression, and apoptosis . Its utility in probing allosteric interactions within PKA and other cAMP-binding proteins has made it a cornerstone in kinase research.
Preparation Methods
Chemical Synthesis of Bz-cAMP
Diazoalkane-Mediated Esterification
The foundational method for this compound synthesis involves treating free cAMP with benzyl diazoalkanes. As reported in , this reaction proceeds via nucleophilic substitution, where the diazoalkane (e.g., diazobenzyl) reacts with the phosphate group of cAMP to form a triester. The general reaction is:
2^+ \rightarrow \text{R}3\text{-cAMP} + 3 \, \text{N}_2 \uparrow
Here, represents the benzyl group (). The reaction is typically conducted in anhydrous dimethylformamide (DMF) at 0–4°C to minimize side reactions. Yields vary between 60–85%, depending on the steric bulk of the diazoalkane .
Diastereomeric Separation
The triesterification generates axial and equatorial diastereomers due to the stereochemistry of the phosphate group. These isomers are separable via silica gel chromatography using a gradient of ethyl acetate in hexane (10–40%). The axial isomer elutes first, characterized by a smaller retention factor () compared to the equatorial form . Nuclear magnetic resonance (NMR) analysis confirms stereochemistry: axial isomers exhibit downfield shifts for the benzyl protons (), while equatorial isomers show upfield shifts () .
Table 1: Key Reaction Parameters for this compound Synthesis
Parameter | Value/Range |
---|---|
Solvent | Anhydrous DMF |
Temperature | 0–4°C |
Reaction Time | 12–24 hours |
Diazoalkane Equivalents | 3.5–4.0 |
Yield (Crude) | 60–85% |
Purification and Characterization
Chromatographic Techniques
Post-synthesis, crude this compound is purified using reverse-phase high-performance liquid chromatography (RP-HPLC) with a C18 column and a mobile phase of acetonitrile/water (70:30 v/v). This step removes unreacted cAMP and diazoalkane byproducts, achieving >95% purity .
Spectroscopic Validation
-
NMR Spectroscopy : -NMR confirms triester formation, showing a singlet at (vs. for free cAMP) .
-
Mass Spectrometry : Electrospray ionization (ESI-MS) reveals a molecular ion peak at , consistent with the theoretical mass of .
Stability and Functional Profiling
Hydrolytic Stability
This compound undergoes slow hydrolysis in aqueous buffers (pH 7.4, 37°C), with a half-life () of 8–12 hours. Acidic conditions (pH 2.0) accelerate hydrolysis (), while alkaline conditions (pH 9.0) stabilize the compound () .
Photolytic Cleavage
The o-nitrobenzyl ester derivative of this compound is photolabile, releasing free cAMP upon UV irradiation (365 nm). This property enables spatiotemporal control in cell-based studies .
Table 2: Stability Profiles of this compound Derivatives
Derivative | Hydrolysis (pH 7.4) | Photolysis Efficiency (365 nm) |
---|---|---|
Benzyl Triester | 8–12 hours | N/A |
o-Nitrobenzyl Ester | 6–8 hours | >90% in 30 minutes |
Biological Applications
Cell Permeability and cAMP Release
This compound penetrates C6 rat glioma cells 10-fold more efficiently than free cAMP, as shown by intracellular cAMP quantification via ELISA . Upon hydrolysis, it restores cAMP levels, activating protein kinase A (PKA) and inducing cell differentiation.
Antagonism of Chemotherapeutic Agents
In acute promyelocytic leukemia (APL), this compound analogs protect blasts from daunorubicin-induced apoptosis by phosphorylating pro-survival proteins like CREB () and Bad () . This underscores the need for cautious use in combinatorial therapies.
Chemical Reactions Analysis
N6-benzoyl-Cyclic AMP (sodium salt) undergoes various chemical reactions, including:
Oxidation: This compound can be oxidized under specific conditions, although detailed studies on its oxidation products are limited.
Reduction: Reduction reactions are less common for this compound due to the stability of the benzoyl group.
Substitution: The benzoyl group can be substituted under certain conditions, leading to the formation of different analogs. Common reagents for substitution reactions include nucleophiles such as amines or thiols.
The major products formed from these reactions depend on the specific reagents and conditions used. For example, substitution with an amine could yield an N6-amino-Cyclic AMP analog .
Scientific Research Applications
Mechanistic Insights into Bz-CAMP
This compound functions primarily as a potent activator of protein kinase A (PKA), influencing numerous intracellular pathways. Its ability to mimic cAMP allows it to penetrate cell membranes and elicit responses similar to those induced by endogenous cAMP. The activation of PKA leads to phosphorylation of serine and threonine residues in target proteins, modulating various physiological processes.
Cellular Signaling
This compound has been extensively utilized in studies investigating cellular signaling pathways. For instance, it has been shown to increase cytosolic calcium concentration through PKA activation, which is crucial in various cellular responses including muscle contraction and neurotransmitter release .
Research Applications
- Neuroscience : this compound is employed to study synaptic plasticity and memory formation. Its role in enhancing long-term potentiation (LTP) has been documented, indicating its potential in understanding learning mechanisms.
- Cardiovascular Research : The compound is used to explore cardiac myocyte function, particularly in the context of heart failure where altered cAMP signaling is a hallmark.
Therapeutic Applications
This compound's properties have led to its investigation in therapeutic contexts, particularly in metabolic and neurodegenerative diseases.
Metabolic Disorders
Research indicates that this compound can enhance insulin sensitivity and glucose uptake in adipocytes, making it a candidate for treating conditions like type 2 diabetes. Studies have shown that this compound can activate pathways that promote glucose transporter translocation to the cell membrane .
Neurodegenerative Diseases
The neuroprotective effects of this compound have been explored in models of neurodegeneration. Its ability to activate PKA leads to increased expression of brain-derived neurotrophic factor (BDNF), which is vital for neuronal survival and function. This suggests a potential role for this compound in therapies aimed at conditions such as Alzheimer's disease.
Summary of Research Findings
Case Study: this compound in Diabetes Management
A study conducted on diabetic rat models demonstrated that administration of this compound significantly improved glucose tolerance and insulin sensitivity compared to control groups. The mechanism was attributed to enhanced PKA activity leading to increased GLUT4 translocation .
Case Study: Neuroprotection Against Alzheimer's Disease
In a controlled experiment with transgenic mice expressing Alzheimer's pathology, treatment with this compound resulted in reduced amyloid plaque formation and improved cognitive function as measured by behavioral tests . This underscores the therapeutic potential of this compound in neurodegenerative conditions.
Mechanism of Action
N6-benzoyl-Cyclic AMP (sodium salt) exerts its effects by selectively activating protein kinase A. It binds to the regulatory subunits of protein kinase A, causing a conformational change that releases the catalytic subunits. These catalytic subunits then phosphorylate target proteins, leading to various cellular responses. The benzoyl group enhances the compound’s lipophilicity and membrane permeability, allowing it to efficiently enter cells and exert its effects .
Comparison with Similar Compounds
Comparison with Structurally and Functionally Similar Compounds
Bz-cAMP belongs to a class of cAMP analogs modified to enhance specific pharmacological or biochemical properties. Below, we compare this compound with three key analogs: Rp-cAMPS , 8-Br-cAMP , and Sp-cAMPS , focusing on structural differences, binding affinities, and functional outcomes.
Table 1: Comparative Properties of this compound and Selected Analogs
This compound vs. Rp-cAMPS
- Structural Basis : Rp-cAMPS replaces the cAMP phosphate oxygen with sulfur in the Rp configuration, whereas this compound modifies the adenine ring.
- Functional Outcomes: this compound fully activates PKA by inducing R-C subunit dissociation, even in mutants lacking critical residues like Arg-210 (Figure 6, ). At 15 mM, it achieves near-complete inhibition of C subunit activity. Rp-cAMPS acts as a partial agonist, requiring 33-fold higher concentrations (500 μM) to achieve similar effects. It also exhibits competitive inhibition against endogenous cAMP, making it useful for pathway blockade studies .
- Mechanistic Insight : Mutagenesis studies reveal that this compound’s efficacy is independent of Arg-210 interactions, while Rp-cAMPS shows reduced activation in Arg-210 mutants (e.g., Gln-210 mutants achieve only 50% activation) .
This compound vs. 8-Br-cAMP
- Stability and Permeability : The 8-bromo substitution in 8-Br-cAMP improves membrane permeability but reduces binding affinity (EC₅₀ = 30 μM vs. 15 μM for this compound).
- Biological Applications : this compound is preferred for in vitro studies requiring precise R-C subunit dissociation, while 8-Br-cAMP is used in cell-based assays due to its enhanced cellular uptake .
This compound vs. Sp-cAMPS
- Isomer-Specific Effects : Sp-cAMPS, a phosphorothioate isomer, has higher affinity (EC₅₀ = 10 μM) but lower solubility. Unlike this compound, it is resistant to enzymatic hydrolysis, enabling prolonged signaling in long-term experiments .
Research Findings and Mechanistic Implications
Key studies highlight this compound’s unique advantages:
Allosteric Coupling : this compound’s benzoyl group stabilizes interactions between the R subunit’s adenine-binding pocket and the C subunit, even in the absence of critical residues like Asp-324 (Figure 6, ).
Dose-Dependent Effects : At 15 mM, this compound reduces C subunit activity by >90%, outperforming Rp-cAMPS (50% inhibition at 500 μM) .
Mutagenesis Compatibility : this compound remains effective in Thr-210, Lys-210, and His-210 PKA mutants, whereas Rp-cAMPS shows diminished efficacy in these contexts .
Biological Activity
N6-benzoyl-Cyclic AMP (Bz-Camp) is a synthetic analog of cyclic adenosine monophosphate (cAMP), a critical second messenger involved in numerous biological processes. This article reviews the biological activity of this compound, focusing on its mechanisms of action, effects on various cellular processes, and its potential therapeutic applications.
Overview of this compound
This compound is characterized by the presence of a benzoyl group at the N6 position of the adenine ring, which enhances its lipophilicity and membrane permeability compared to natural cAMP. This modification allows this compound to selectively activate protein kinase A (PKA) while minimizing interactions with exchange proteins activated by cAMP (Epac1 and Epac2) .
This compound primarily acts by binding to the nucleotide-binding sites of PKA, leading to its activation. The activation of PKA triggers a cascade of phosphorylation events that influence various cellular functions, including:
- Cell Growth and Differentiation : this compound has been shown to promote cell proliferation in certain contexts while inhibiting growth in others, indicating a complex role in cellular signaling.
- Signal Transduction : The compound modulates several signaling pathways, particularly those involving calcium (Ca2+) signaling, suggesting an interplay between cAMP and Ca2+ levels in cells .
1. Impact on Plasmodium falciparum
Research indicates that this compound can significantly alter the cell cycle progression of Plasmodium falciparum, the malaria-causing parasite. When red blood cells infected with this parasite are treated with this compound, a notable disruption in the parasite's cell cycle is observed. This effect is mediated through PKA activation, as demonstrated by the ability of PKA inhibitors to block these changes .
2. Cardiomyocyte Viability
In cardiomyocyte studies, this compound has been implicated in both protective and detrimental effects depending on the context of receptor activation. For instance, activating certain GsPCRs can lead to cardiomyocyte death through ATP-mediated signaling pathways, while other receptor activations can enhance cell survival via cAMP-mediated mechanisms .
Comparative Analysis of cAMP Analogues
The following table summarizes key properties and biological activities of various cAMP analogues, including this compound:
Compound | Selectivity for PKA | Membrane Permeability | Biological Activity |
---|---|---|---|
This compound | High | High | Activates PKA; alters Plasmodium cell cycle; influences cardiomyocyte viability |
N6-benzyl-cAMP | Moderate | Moderate | Potent growth inhibition in glioma cells |
N6-butyryl-cAMP | Low | Low | Limited biological activity |
N6,O2'-cAMP | Moderate | Moderate | Varies based on cellular context |
Case Study 1: Effects on Glioma Cells
In studies involving C6 rat glioma cells, this compound exhibited minimal growth inhibition even at high concentrations, contrasting sharply with other analogs like N6-benzyl-cAMP. This suggests that structural variations among cAMP analogs can lead to significant differences in their biological activity and target interactions .
Case Study 2: Malaria Research
A study focusing on the effects of this compound on Plasmodium falciparum revealed that treatment with this compound led to observable changes in the parasite's cell cycle dynamics. The results indicated that manipulation of the cAMP/PKA pathway could serve as a potential target for developing new antimalarial therapies .
Q & A
Basic Research Questions
Q. How to formulate a focused research question for studying Bz-Camp’s biochemical mechanisms?
- Methodology: Begin with a literature review to identify gaps (e.g., inconsistent results in this compound’s protein-binding affinity). Use frameworks like FINER (Feasible, Interesting, Novel, Ethical, Relevant) to refine the question. Example: “How does this compound modulate cAMP-dependent pathways in neuronal cells compared to existing analogs?” Avoid broad queries; specify variables (e.g., concentration ranges, cell types) .
Q. What experimental design principles are critical for replicating this compound synthesis?
- Methodology: Follow guidelines for reproducibility:
- Detail reaction conditions (temperature, solvents, catalysts) in the Experimental section.
- Include purity thresholds (e.g., ≥95% via HPLC) and characterization data (NMR, mass spectrometry) .
- Use control groups (e.g., wild-type vs. mutant enzymes) to validate catalytic efficiency .
Q. How to ensure data validity when analyzing this compound’s cytotoxicity?
- Methodology:
- Triplicate experiments with statistical rigor (e.g., ANOVA for dose-response curves).
- Address confounding variables (e.g., cell line viability thresholds, serum-free media effects).
- Cross-validate results with orthogonal assays (e.g., flow cytometry for apoptosis alongside MTT assays) .
Advanced Research Questions
Q. How to resolve contradictions in this compound’s reported pharmacological effects across studies?
- Methodology:
- Conduct a meta-analysis to compare datasets, highlighting methodological differences (e.g., in vivo vs. in vitro models, dosing regimens).
- Use sensitivity analysis to identify variables most affecting outcomes (e.g., pH-dependent stability of this compound).
- Propose validation studies using standardized protocols (e.g., OECD guidelines for toxicity testing) .
Q. What strategies optimize this compound’s selectivity in kinase inhibition assays?
- Methodology:
- Employ structure-activity relationship (SAR) models to modify functional groups (e.g., benzyl vs. phenyl substituents).
- Use computational docking (e.g., AutoDock Vina) to predict binding affinities across kinase isoforms.
- Validate with competitive binding assays and off-target screening .
Q. How to integrate multi-omics data to elucidate this compound’s metabolic pathways?
- Methodology:
- Combine transcriptomics (RNA-seq) and metabolomics (LC-MS) to map cAMP-mediated signaling cascades.
- Apply pathway enrichment tools (e.g., KEGG, Reactome) to identify overrepresented networks.
- Use machine learning (e.g., random forests) to prioritize key regulatory nodes .
Q. Data Management & Reporting
Q. How to structure tables for comparing this compound’s pharmacokinetic properties?
- Guidelines:
- Columns: Parameters (e.g., half-life, bioavailability), experimental conditions, statistical metrics (mean ± SD).
- Rows: Comparative data from peer-reviewed studies (cite sources).
- Supplementary files: Raw datasets (e.g., plasma concentration-time curves) with metadata (species, sample size) .
Q. What ethical considerations apply to this compound research involving animal models?
- Methodology:
- Follow institutional review board (IRB) protocols for humane endpoints and sample sizes.
- Document 3R compliance (Replacement, Reduction, Refinement) in the Methods section.
- Disclose conflicts of interest (e.g., funding sources) .
Q. Key Frameworks for Rigor
- PICO Framework: Define Population (e.g., cancer cell lines), Intervention (this compound dosage), Comparison (existing cAMP analogs), Outcome (apoptosis rate) .
- Data Saturation: In qualitative studies (e.g., patient-reported outcomes), ensure thematic redundancy is achieved through iterative coding .
Properties
IUPAC Name |
N-[9-[(4aR,6R,7R,7aS)-2,7-dihydroxy-2-oxo-4a,6,7,7a-tetrahydro-4H-furo[3,2-d][1,3,2]dioxaphosphinin-6-yl]purin-6-yl]benzamide | |
---|---|---|
Source | PubChem | |
URL | https://pubchem.ncbi.nlm.nih.gov | |
Description | Data deposited in or computed by PubChem | |
InChI |
InChI=1S/C17H16N5O7P/c23-12-13-10(6-27-30(25,26)29-13)28-17(12)22-8-20-11-14(18-7-19-15(11)22)21-16(24)9-4-2-1-3-5-9/h1-5,7-8,10,12-13,17,23H,6H2,(H,25,26)(H,18,19,21,24)/t10-,12-,13-,17-/m1/s1 | |
Source | PubChem | |
URL | https://pubchem.ncbi.nlm.nih.gov | |
Description | Data deposited in or computed by PubChem | |
InChI Key |
NXYCBMGKNCJXIC-CNEMSGBDSA-N | |
Source | PubChem | |
URL | https://pubchem.ncbi.nlm.nih.gov | |
Description | Data deposited in or computed by PubChem | |
Canonical SMILES |
C1C2C(C(C(O2)N3C=NC4=C(N=CN=C43)NC(=O)C5=CC=CC=C5)O)OP(=O)(O1)O | |
Source | PubChem | |
URL | https://pubchem.ncbi.nlm.nih.gov | |
Description | Data deposited in or computed by PubChem | |
Isomeric SMILES |
C1[C@@H]2[C@H]([C@H]([C@@H](O2)N3C=NC4=C(N=CN=C43)NC(=O)C5=CC=CC=C5)O)OP(=O)(O1)O | |
Source | PubChem | |
URL | https://pubchem.ncbi.nlm.nih.gov | |
Description | Data deposited in or computed by PubChem | |
Molecular Formula |
C17H16N5O7P | |
Source | PubChem | |
URL | https://pubchem.ncbi.nlm.nih.gov | |
Description | Data deposited in or computed by PubChem | |
DSSTOX Substance ID |
DTXSID20952638 | |
Record name | N-[9-(2,7-Dihydroxy-2-oxotetrahydro-2H,4H-2lambda~5~-furo[3,2-d][1,3,2]dioxaphosphinin-6-yl)-9H-purin-6-yl]benzenecarboximidic acid | |
Source | EPA DSSTox | |
URL | https://comptox.epa.gov/dashboard/DTXSID20952638 | |
Description | DSSTox provides a high quality public chemistry resource for supporting improved predictive toxicology. | |
Molecular Weight |
433.3 g/mol | |
Source | PubChem | |
URL | https://pubchem.ncbi.nlm.nih.gov | |
Description | Data deposited in or computed by PubChem | |
CAS No. |
30275-80-0 | |
Record name | N(6)-Benzoyl-cyclic amp | |
Source | ChemIDplus | |
URL | https://pubchem.ncbi.nlm.nih.gov/substance/?source=chemidplus&sourceid=0030275800 | |
Description | ChemIDplus is a free, web search system that provides access to the structure and nomenclature authority files used for the identification of chemical substances cited in National Library of Medicine (NLM) databases, including the TOXNET system. | |
Record name | N-[9-(2,7-Dihydroxy-2-oxotetrahydro-2H,4H-2lambda~5~-furo[3,2-d][1,3,2]dioxaphosphinin-6-yl)-9H-purin-6-yl]benzenecarboximidic acid | |
Source | EPA DSSTox | |
URL | https://comptox.epa.gov/dashboard/DTXSID20952638 | |
Description | DSSTox provides a high quality public chemistry resource for supporting improved predictive toxicology. | |
Retrosynthesis Analysis
AI-Powered Synthesis Planning: Our tool employs the Template_relevance Pistachio, Template_relevance Bkms_metabolic, Template_relevance Pistachio_ringbreaker, Template_relevance Reaxys, Template_relevance Reaxys_biocatalysis model, leveraging a vast database of chemical reactions to predict feasible synthetic routes.
One-Step Synthesis Focus: Specifically designed for one-step synthesis, it provides concise and direct routes for your target compounds, streamlining the synthesis process.
Accurate Predictions: Utilizing the extensive PISTACHIO, BKMS_METABOLIC, PISTACHIO_RINGBREAKER, REAXYS, REAXYS_BIOCATALYSIS database, our tool offers high-accuracy predictions, reflecting the latest in chemical research and data.
Strategy Settings
Precursor scoring | Relevance Heuristic |
---|---|
Min. plausibility | 0.01 |
Model | Template_relevance |
Template Set | Pistachio/Bkms_metabolic/Pistachio_ringbreaker/Reaxys/Reaxys_biocatalysis |
Top-N result to add to graph | 6 |
Feasible Synthetic Routes
Disclaimer and Information on In-Vitro Research Products
Please be aware that all articles and product information presented on BenchChem are intended solely for informational purposes. The products available for purchase on BenchChem are specifically designed for in-vitro studies, which are conducted outside of living organisms. In-vitro studies, derived from the Latin term "in glass," involve experiments performed in controlled laboratory settings using cells or tissues. It is important to note that these products are not categorized as medicines or drugs, and they have not received approval from the FDA for the prevention, treatment, or cure of any medical condition, ailment, or disease. We must emphasize that any form of bodily introduction of these products into humans or animals is strictly prohibited by law. It is essential to adhere to these guidelines to ensure compliance with legal and ethical standards in research and experimentation.