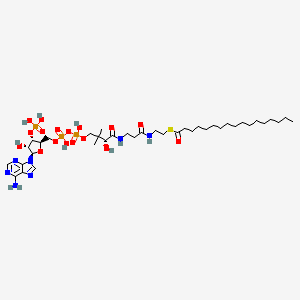
Heptadecanoyl-coa
Overview
Description
Heptadecanoyl Coenzyme A is a long-chain saturated fatty acyl-Coenzyme A. It plays a crucial role as an intermediate in lipid metabolism. This compound is involved in various biochemical processes, particularly within the mitochondria and cytoplasm, where it acts as a substrate for specific enzymes .
Mechanism of Action
Heptadecanoyl-coa, also known as Heptadecyl-coa, is a long-chain fatty acyl-CoA that plays a crucial role in lipid metabolism . This article aims to provide a comprehensive overview of the mechanism of action of this compound.
Target of Action
this compound primarily targets two enzymes: acyl-CoA dehydrogenase within the mitochondria and carnitine O-palmitoyltransferase within the cytoplasm . These enzymes play a significant role in the metabolism of fatty acids.
Mode of Action
this compound interacts with its targets by serving as a substrate. It is used as an intermediate in lipid metabolism, where it undergoes enzymatic reactions facilitated by acyl-CoA dehydrogenase and carnitine O-palmitoyltransferase . Moreover, this compound can inhibit general acyl-coenzyme A dehydrogenase .
Biochemical Pathways
this compound is involved in the fatty acid metabolic pathway. As a substrate of acyl-CoA dehydrogenase and carnitine O-palmitoyltransferase, it participates in the breakdown of fatty acids, contributing to energy production within the cell .
Result of Action
The action of this compound results in the breakdown of fatty acids, contributing to energy production within the cell . This process is crucial for maintaining cellular functions and overall energy balance.
Biochemical Analysis
Biochemical Properties
Heptadecanoyl-coenzyme A functions as an acyl group carrier in lipid metabolism. It is a substrate for several enzymes, including acyl-coenzyme A dehydrogenase and carnitine O-palmitoyltransferase . Acyl-coenzyme A dehydrogenase catalyzes the initial step in the mitochondrial beta-oxidation of fatty acids, converting heptadecanoyl-coenzyme A into enoyl-coenzyme A. Carnitine O-palmitoyltransferase facilitates the transport of heptadecanoyl-coenzyme A into the mitochondria for further oxidation . Additionally, heptadecanoyl-coenzyme A acts as an inhibitor of general acyl-coenzyme A dehydrogenase .
Cellular Effects
Heptadecanoyl-coenzyme A influences various cellular processes, including energy production, lipid synthesis, and signal transduction. In energy metabolism, it undergoes beta-oxidation to produce acetyl-coenzyme A, which enters the citric acid cycle to generate ATP . In lipid synthesis, heptadecanoyl-coenzyme A serves as a precursor for the synthesis of complex lipids, such as phospholipids and triglycerides . It also plays a role in regulating gene expression by modulating the activity of transcription factors involved in lipid metabolism .
Molecular Mechanism
At the molecular level, heptadecanoyl-coenzyme A exerts its effects through binding interactions with various enzymes and proteins. It binds to acyl-coenzyme A dehydrogenase, facilitating the dehydrogenation of fatty acids . It also interacts with carnitine O-palmitoyltransferase, enabling the transport of fatty acids into the mitochondria . Additionally, heptadecanoyl-coenzyme A can modulate gene expression by influencing the activity of transcription factors involved in lipid metabolism .
Temporal Effects in Laboratory Settings
In laboratory settings, the stability and degradation of heptadecanoyl-coenzyme A can vary over time. Studies have shown that heptadecanoyl-coenzyme A is relatively stable at low temperatures but may degrade at higher temperatures . Long-term exposure to heptadecanoyl-coenzyme A can lead to changes in cellular function, including alterations in lipid metabolism and energy production . These effects are observed in both in vitro and in vivo studies .
Dosage Effects in Animal Models
The effects of heptadecanoyl-coenzyme A vary with different dosages in animal models. At low doses, heptadecanoyl-coenzyme A supports normal lipid metabolism and energy production . At high doses, it can lead to toxic effects, including liver dysfunction and oxidative stress . Threshold effects have been observed, where a certain dosage is required to elicit a significant biological response .
Metabolic Pathways
Heptadecanoyl-coenzyme A is involved in several metabolic pathways, including beta-oxidation and lipid synthesis. In beta-oxidation, it is converted into enoyl-coenzyme A by acyl-coenzyme A dehydrogenase . In lipid synthesis, it serves as a precursor for the synthesis of complex lipids, such as phospholipids and triglycerides . The enzyme carnitine O-palmitoyltransferase plays a crucial role in transporting heptadecanoyl-coenzyme A into the mitochondria for further oxidation .
Transport and Distribution
Heptadecanoyl-coenzyme A is transported and distributed within cells and tissues through interactions with specific transporters and binding proteins. Carnitine O-palmitoyltransferase facilitates the transport of heptadecanoyl-coenzyme A into the mitochondria . Within the mitochondria, it undergoes beta-oxidation to produce acetyl-coenzyme A . The distribution of heptadecanoyl-coenzyme A within cells is influenced by its interactions with various binding proteins .
Subcellular Localization
Heptadecanoyl-coenzyme A is localized in different subcellular compartments, including the cytoplasm and mitochondria. In the cytoplasm, it is involved in lipid synthesis and signal transduction . In the mitochondria, it undergoes beta-oxidation to produce acetyl-coenzyme A . The subcellular localization of heptadecanoyl-coenzyme A is regulated by targeting signals and post-translational modifications that direct it to specific compartments or organelles .
Preparation Methods
Synthetic Routes and Reaction Conditions: Heptadecanoyl Coenzyme A can be synthesized through the formal condensation of the thiol group of coenzyme A with the carboxy group of heptadecanoic acid . The reaction typically involves the use of activating agents to facilitate the formation of the thioester bond.
Industrial Production Methods: Industrial production of Heptadecanoyl Coenzyme A often involves enzymatic synthesis using acyl-CoA synthetase enzymes. These enzymes catalyze the formation of acyl-CoA from fatty acids, coenzyme A, and ATP under specific conditions .
Types of Reactions:
Reduction: It can be reduced to its corresponding alcohol under specific conditions.
Substitution: The compound can participate in substitution reactions where the acyl group is transferred to other molecules.
Common Reagents and Conditions:
Oxidation: Requires acyl-CoA dehydrogenase and oxygen.
Reduction: Typically involves reducing agents such as sodium borohydride.
Substitution: Requires nucleophiles and appropriate catalysts.
Major Products:
Oxidation: Produces enoyl-CoA and subsequently acetyl-CoA.
Reduction: Produces heptadecanol.
Substitution: Produces various acylated compounds depending on the nucleophile used.
Scientific Research Applications
Heptadecanoyl Coenzyme A has diverse applications in scientific research:
Chemistry: Used as an intermediate in the synthesis of complex lipids and other biochemical compounds.
Biology: Plays a role in studying lipid metabolism and enzyme kinetics.
Medicine: Investigated for its potential role in metabolic disorders and as a biomarker for certain diseases.
Industry: Utilized in the production of biofuels and biodegradable plastics.
Comparison with Similar Compounds
Acetyl Coenzyme A: A short-chain acyl-CoA involved in the tricarboxylic acid cycle.
Oleoyl Coenzyme A: A long-chain unsaturated fatty acyl-CoA involved in lipid metabolism.
Palmitoyl Coenzyme A: Another long-chain saturated fatty acyl-CoA with similar metabolic roles.
Uniqueness: Heptadecanoyl Coenzyme A is unique due to its specific chain length (C-17), which influences its interaction with enzymes and its role in lipid metabolism. Its inhibitory effect on general acyl-coenzyme A dehydrogenase also sets it apart from other acyl-CoAs .
Biological Activity
Heptadecanoyl-CoA, a long-chain fatty acyl-CoA, plays a significant role in various metabolic pathways, particularly in lipid metabolism and energy homeostasis. This article explores its biological activity, focusing on its metabolic functions, implications in disease, and potential as a biomarker.
This compound (C17:0) is formed by the condensation of coenzyme A (CoA) with heptadecanoic acid. Its chemical formula is . This compound is crucial for various biochemical processes, including fatty acid metabolism and energy production.
Metabolic Role
This compound is involved in several metabolic pathways:
- Fatty Acid β-Oxidation : It serves as a substrate for β-oxidation, a process where fatty acids are broken down to generate acetyl-CoA, which enters the citric acid cycle for energy production.
- Lipid Synthesis : this compound can be used in the synthesis of complex lipids, contributing to membrane formation and cellular signaling.
- Acylation Reactions : It participates in acylation reactions catalyzed by various acyl-CoA transferases, influencing lipid composition in membranes .
1. Biomarker Potential
Recent studies have identified heptadecanoylcarnitine (C17) as a novel biomarker for certain metabolic disorders such as propionic acidemia (PA) and methylmalonic acidemia (MMA). In a cohort study involving neonates diagnosed through newborn screening programs, elevated levels of heptadecanoylcarnitine were significantly associated with these conditions. Specifically, 21 out of 23 neonates with MMA showed higher levels compared to controls, indicating its potential use in early diagnosis .
Condition | Number of Cases | Elevated C17 Levels |
---|---|---|
MMA | 22 | Yes |
PA | 1 | Yes |
2. Influence on Lipid Metabolism
This compound's role in lipid metabolism was highlighted in a study examining the effects of Nudt7 overexpression on hepatic CoA levels. The research demonstrated that altering the concentration of various acyl-CoAs, including this compound, affected bile acid synthesis and fatty acid oxidation rates in mouse models. Specifically, overexpression led to decreased concentrations of short-chain acyl-CoAs and choloyl-CoA while increasing medium- and long-chain acylcarnitines .
Implications in Health and Disease
The accumulation of this compound has been linked to metabolic dysregulation. Elevated levels can indicate disturbances in fatty acid metabolism, which may contribute to conditions such as obesity and insulin resistance. For instance, studies have shown that alterations in long-chain acyl-CoAs can affect thermogenic responses in adipose tissues, influencing overall energy expenditure .
Properties
IUPAC Name |
S-[2-[3-[[(2R)-4-[[[(2R,3S,4R,5R)-5-(6-aminopurin-9-yl)-4-hydroxy-3-phosphonooxyoxolan-2-yl]methoxy-hydroxyphosphoryl]oxy-hydroxyphosphoryl]oxy-2-hydroxy-3,3-dimethylbutanoyl]amino]propanoylamino]ethyl] heptadecanethioate | |
---|---|---|
Source | PubChem | |
URL | https://pubchem.ncbi.nlm.nih.gov | |
Description | Data deposited in or computed by PubChem | |
InChI |
InChI=1S/C38H68N7O17P3S/c1-4-5-6-7-8-9-10-11-12-13-14-15-16-17-18-29(47)66-22-21-40-28(46)19-20-41-36(50)33(49)38(2,3)24-59-65(56,57)62-64(54,55)58-23-27-32(61-63(51,52)53)31(48)37(60-27)45-26-44-30-34(39)42-25-43-35(30)45/h25-27,31-33,37,48-49H,4-24H2,1-3H3,(H,40,46)(H,41,50)(H,54,55)(H,56,57)(H2,39,42,43)(H2,51,52,53)/t27-,31-,32-,33+,37-/m1/s1 | |
Source | PubChem | |
URL | https://pubchem.ncbi.nlm.nih.gov | |
Description | Data deposited in or computed by PubChem | |
InChI Key |
DRABUZIHHACUPI-DUPKZGIXSA-N | |
Source | PubChem | |
URL | https://pubchem.ncbi.nlm.nih.gov | |
Description | Data deposited in or computed by PubChem | |
Canonical SMILES |
CCCCCCCCCCCCCCCCC(=O)SCCNC(=O)CCNC(=O)C(C(C)(C)COP(=O)(O)OP(=O)(O)OCC1C(C(C(O1)N2C=NC3=C(N=CN=C32)N)O)OP(=O)(O)O)O | |
Source | PubChem | |
URL | https://pubchem.ncbi.nlm.nih.gov | |
Description | Data deposited in or computed by PubChem | |
Isomeric SMILES |
CCCCCCCCCCCCCCCCC(=O)SCCNC(=O)CCNC(=O)[C@@H](C(C)(C)COP(=O)(O)OP(=O)(O)OC[C@@H]1[C@H]([C@H]([C@@H](O1)N2C=NC3=C(N=CN=C32)N)O)OP(=O)(O)O)O | |
Source | PubChem | |
URL | https://pubchem.ncbi.nlm.nih.gov | |
Description | Data deposited in or computed by PubChem | |
Molecular Formula |
C38H68N7O17P3S | |
Source | PubChem | |
URL | https://pubchem.ncbi.nlm.nih.gov | |
Description | Data deposited in or computed by PubChem | |
DSSTOX Substance ID |
DTXSID201316404 | |
Record name | Heptadecanoyl-CoA | |
Source | EPA DSSTox | |
URL | https://comptox.epa.gov/dashboard/DTXSID201316404 | |
Description | DSSTox provides a high quality public chemistry resource for supporting improved predictive toxicology. | |
Molecular Weight |
1020.0 g/mol | |
Source | PubChem | |
URL | https://pubchem.ncbi.nlm.nih.gov | |
Description | Data deposited in or computed by PubChem | |
Physical Description |
Solid | |
Record name | Heptadecanoyl CoA | |
Source | Human Metabolome Database (HMDB) | |
URL | http://www.hmdb.ca/metabolites/HMDB0006497 | |
Description | The Human Metabolome Database (HMDB) is a freely available electronic database containing detailed information about small molecule metabolites found in the human body. | |
Explanation | HMDB is offered to the public as a freely available resource. Use and re-distribution of the data, in whole or in part, for commercial purposes requires explicit permission of the authors and explicit acknowledgment of the source material (HMDB) and the original publication (see the HMDB citing page). We ask that users who download significant portions of the database cite the HMDB paper in any resulting publications. | |
CAS No. |
3546-17-6 | |
Record name | Heptadecanoyl-CoA | |
Source | CAS Common Chemistry | |
URL | https://commonchemistry.cas.org/detail?cas_rn=3546-17-6 | |
Description | CAS Common Chemistry is an open community resource for accessing chemical information. Nearly 500,000 chemical substances from CAS REGISTRY cover areas of community interest, including common and frequently regulated chemicals, and those relevant to high school and undergraduate chemistry classes. This chemical information, curated by our expert scientists, is provided in alignment with our mission as a division of the American Chemical Society. | |
Explanation | The data from CAS Common Chemistry is provided under a CC-BY-NC 4.0 license, unless otherwise stated. | |
Record name | Heptadecanoyl-coenzyme A | |
Source | ChemIDplus | |
URL | https://pubchem.ncbi.nlm.nih.gov/substance/?source=chemidplus&sourceid=0003546176 | |
Description | ChemIDplus is a free, web search system that provides access to the structure and nomenclature authority files used for the identification of chemical substances cited in National Library of Medicine (NLM) databases, including the TOXNET system. | |
Record name | Heptadecanoyl-CoA | |
Source | EPA DSSTox | |
URL | https://comptox.epa.gov/dashboard/DTXSID201316404 | |
Description | DSSTox provides a high quality public chemistry resource for supporting improved predictive toxicology. | |
Record name | Heptadecanoyl CoA | |
Source | Human Metabolome Database (HMDB) | |
URL | http://www.hmdb.ca/metabolites/HMDB0006497 | |
Description | The Human Metabolome Database (HMDB) is a freely available electronic database containing detailed information about small molecule metabolites found in the human body. | |
Explanation | HMDB is offered to the public as a freely available resource. Use and re-distribution of the data, in whole or in part, for commercial purposes requires explicit permission of the authors and explicit acknowledgment of the source material (HMDB) and the original publication (see the HMDB citing page). We ask that users who download significant portions of the database cite the HMDB paper in any resulting publications. | |
Retrosynthesis Analysis
AI-Powered Synthesis Planning: Our tool employs the Template_relevance Pistachio, Template_relevance Bkms_metabolic, Template_relevance Pistachio_ringbreaker, Template_relevance Reaxys, Template_relevance Reaxys_biocatalysis model, leveraging a vast database of chemical reactions to predict feasible synthetic routes.
One-Step Synthesis Focus: Specifically designed for one-step synthesis, it provides concise and direct routes for your target compounds, streamlining the synthesis process.
Accurate Predictions: Utilizing the extensive PISTACHIO, BKMS_METABOLIC, PISTACHIO_RINGBREAKER, REAXYS, REAXYS_BIOCATALYSIS database, our tool offers high-accuracy predictions, reflecting the latest in chemical research and data.
Strategy Settings
Precursor scoring | Relevance Heuristic |
---|---|
Min. plausibility | 0.01 |
Model | Template_relevance |
Template Set | Pistachio/Bkms_metabolic/Pistachio_ringbreaker/Reaxys/Reaxys_biocatalysis |
Top-N result to add to graph | 6 |
Feasible Synthetic Routes
Q1: How does Heptadecyl-CoA interact with enzymes, and what are the downstream effects?
A: Heptadecyl-CoA acts as a potent competitive inhibitor of pig kidney general acyl-CoA dehydrogenase. [] This enzyme, crucial for fatty acid oxidation, is inhibited by Heptadecyl-CoA's strong binding at the substrate binding site, preventing the natural substrate, octanoyl-CoA, from binding. [] This interaction highlights Heptadecyl-CoA's potential as a tool to investigate fatty acid metabolism.
Q2: What are the catalytic properties of structurally similar compounds like Heptadecan-2-onyldethio-CoA, and what are their applications?
A: Heptadecan-2-onyldethio-CoA, a carba analog of palmitoyl-CoA, serves as a substrate for pig kidney general acyl-CoA dehydrogenase, albeit with lower dehydrogenation efficiency compared to palmitoyl-CoA. [] This suggests that the enzyme's active site can accommodate modifications at the thioester linkage, indicating flexibility in substrate recognition. This finding further supports the hypothesis that the dehydrogenation mechanism involves an alpha-carbanion intermediate. [] Such analogs are valuable for dissecting enzymatic mechanisms and exploring substrate specificity.
Q3: Can you elaborate on the structural characteristics of Heptadecyl-CoA?
A3: While specific spectroscopic data is not provided in the research excerpts, Heptadecyl-CoA's structure can be understood by its name. As a thioester, it consists of a 17-carbon saturated fatty acid (heptadecanoic acid) linked to coenzyme A through a thioester bond. Coenzyme A is a crucial molecule involved in various metabolic pathways, particularly those concerning fatty acids.
Q4: Are there any research efforts focused on the in vivo effects of Heptadecyl-CoA or its analogs?
A: While the provided excerpts primarily focus on in vitro studies, research using [2-(14)C] acetate in decapitated females of the moth Heliothis virescens suggests that neuropeptide PBAN might regulate enzymes involved in fatty acid synthesis, such as acetyl CoA carboxylase, impacting the availability of precursors for Heptadecyl-CoA synthesis. [] This indirect evidence highlights the potential regulatory roles of compounds like Heptadecyl-CoA in complex biological processes like pheromone biosynthesis.
Disclaimer and Information on In-Vitro Research Products
Please be aware that all articles and product information presented on BenchChem are intended solely for informational purposes. The products available for purchase on BenchChem are specifically designed for in-vitro studies, which are conducted outside of living organisms. In-vitro studies, derived from the Latin term "in glass," involve experiments performed in controlled laboratory settings using cells or tissues. It is important to note that these products are not categorized as medicines or drugs, and they have not received approval from the FDA for the prevention, treatment, or cure of any medical condition, ailment, or disease. We must emphasize that any form of bodily introduction of these products into humans or animals is strictly prohibited by law. It is essential to adhere to these guidelines to ensure compliance with legal and ethical standards in research and experimentation.