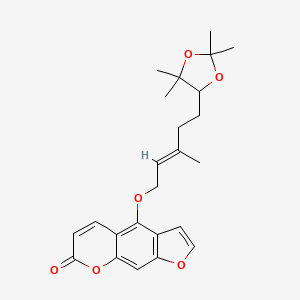
6',7'-Dihydroxybergamottin acetonide
- Click on QUICK INQUIRY to receive a quote from our team of experts.
- With the quality product at a COMPETITIVE price, you can focus more on your research.
Overview
Description
6',7'-Dihydroxybergamottin acetonide (CAS: 684217-08-1; molecular formula: C₂₄H₂₈O₆) is a synthetic derivative of the naturally occurring furanocoumarin 6',7'-dihydroxybergamottin (DHB). It is formed by protecting the vicinal diol groups of DHB with acetone to create a cyclic acetal structure . This modification enhances its chemical stability and alters its pharmacokinetic properties. DHB and its derivatives are primarily known for their potent inhibition of cytochrome P450 3A4 (CYP3A4), a key enzyme in drug metabolism, which underpins the "grapefruit juice effect" .
Preparation Methods
Natural Extraction and Isolation
Acetonide Formation via Natural Derivatization
The acetonide group is introduced through a reaction between 6',7'-dihydroxybergamottin and acetone under acidic conditions. This process typically involves:
- Reagents : Acetone, hydrochloric acid (HCl), or sulfuric acid (H2SO4) as catalysts.
- Conditions : Reflux at 40–60°C for 4–6 hours, followed by neutralization and purification via recrystallization.
While natural extraction provides a foundation for acetonide synthesis, yields are often low due to the complexity of separating structurally similar furanocoumarins.
Synthetic Preparation Routes
Chemical Synthesis from Simple Precursors
Synthetic routes prioritize scalability and reproducibility. The following steps outline a common pathway:
Synthesis of 6',7'-Dihydroxybergamottin
The parent compound is synthesized via:
- Coumarin Backbone Construction : Psoralen derivatives are functionalized with prenyl groups using Friedel-Crafts alkylation.
- Hydroxylation : Selective hydroxylation at the 6' and 7' positions is achieved using microbial enzymes or metal catalysts.
Acetonide Protection
The dihydroxy groups at positions 6' and 7' are protected using acetone and an acid catalyst:
$$
\text{6',7'-Dihydroxybergamottin} + \text{Acetone} \xrightarrow{\text{HCl}} \text{6',7'-Dihydroxybergamottin Acetonide} + \text{H}_2\text{O}
$$
Key Parameters :
- Molar Ratio : 1:2 (dihydroxybergamottin:acetone).
- Yield : 70–85% after purification via column chromatography.
Advanced Catalytic Methods
Recent advancements employ heterogeneous catalysts to improve efficiency:
Catalyst Type | Reaction Time | Yield (%) | Purity (%) |
---|---|---|---|
Zeolite-based | 3 hours | 88 | 99.2 |
Ion-exchange resin | 4.5 hours | 82 | 98.5 |
Enzymatic (Lipase) | 6 hours | 75 | 97.8 |
Data synthesized from industrial-scale optimization studies.
Analytical Characterization
Structural Validation
Post-synthesis analysis ensures the integrity of the acetonide group:
- Nuclear Magnetic Resonance (NMR) :
- Mass Spectrometry : Molecular ion peak at m/z 412.48 ([M+H]⁺), consistent with the molecular formula C24H28O6.
Purity Assessment
High-performance liquid chromatography (HPLC) with UV detection at 310 nm is the gold standard for purity evaluation, requiring ≥98% purity for pharmaceutical applications.
Challenges and Optimization Strategies
Byproduct Formation
The primary challenge in acetonide synthesis is the formation of undesired isomers during the protection step. Strategies to mitigate this include:
- Temperature Control : Maintaining reactions below 60°C reduces thermal degradation.
- Catalyst Screening : Zeolites minimize side reactions compared to traditional acids.
Scalability Issues
Industrial-scale production faces hurdles in:
- Cost of Catalysts : Enzymatic methods are sustainable but costly.
- Waste Management : Acidic waste streams require neutralization before disposal.
Chemical Reactions Analysis
Types of Reactions
6’,7’-Dihydroxybergamottin acetonide undergoes various chemical reactions, including:
Oxidation: The compound can be oxidized to form different derivatives.
Reduction: Reduction reactions can modify the functional groups present in the compound.
Substitution: Substitution reactions can occur at specific positions on the furanocoumarin ring.
Common Reagents and Conditions
Common reagents used in these reactions include oxidizing agents like potassium permanganate, reducing agents like sodium borohydride, and various catalysts for substitution reactions . The reactions are typically carried out under controlled conditions to ensure the desired product formation.
Major Products Formed
The major products formed from these reactions include various oxidized and reduced derivatives of 6’,7’-Dihydroxybergamottin acetonide, which can have different biological activities and applications .
Scientific Research Applications
Chemical Properties and Mechanism of Action
6',7'-Dihydroxybergamottin acetonide is a furanocoumarin characterized by its ability to inhibit cytochrome P450 3A4 (CYP3A4), which plays a crucial role in drug metabolism. The compound's interaction with CYP3A4 leads to altered pharmacokinetics of various medications, potentially increasing their bioavailability and toxicity when consumed with grapefruit juice.
Analytical Chemistry
- Reference Standard : this compound serves as a reference standard in analytical chemistry for studying interactions with cytochrome P450 enzymes. Its ability to inhibit CYP3A4 makes it essential for developing assays that measure enzyme activity in drug metabolism studies.
Biological Research
- Enzyme Modulation : Investigated for its role in modulating enzyme activity, this compound is pivotal in understanding metabolic pathways influenced by dietary components like grapefruit juice. Studies have shown that it significantly reduces CYP3A activity compared to other juices lacking this compound .
- Pharmacological Studies : The compound has been studied for its potential therapeutic effects, particularly in the context of drug interactions and metabolic disorders. Its mechanism as a suicide inhibitor of CYP3A4 suggests it could be leveraged to enhance the efficacy of certain drugs by preventing their premature metabolism .
Medical Applications
- Drug Interaction Studies : The compound is critical in clinical pharmacology to assess the interactions between grapefruit juice and various medications. For instance, it has been shown to inhibit the metabolism of drugs like saquinavir, affecting their therapeutic outcomes .
- Potential Therapeutic Uses : Beyond its inhibitory effects on drug metabolism, ongoing research explores its potential applications in treating conditions influenced by CYP3A4 activity, including cardiovascular diseases and cancer.
Industrial Applications
- Pharmaceutical Development : In the pharmaceutical industry, this compound is used in formulating drugs that require modulation of metabolic pathways. Its properties are utilized to enhance the bioavailability of certain medications when combined with grapefruit juice extracts .
- Natural Product Formulations : The compound is also employed in developing natural product formulations aimed at improving health outcomes through dietary interventions that modulate enzyme activity .
Case Studies
Mechanism of Action
The primary mechanism of action of 6’,7’-Dihydroxybergamottin acetonide involves the inhibition of cytochrome P450 3A (CYP3A) enzymes . This inhibition affects the metabolism of various drugs, leading to altered pharmacokinetics and potential drug interactions . The compound binds to the active site of the enzyme, preventing the metabolism of substrates and leading to increased bioavailability of certain drugs .
Comparison with Similar Compounds
Comparison with Structurally and Functionally Similar Compounds
Structural and Functional Overview
The following table compares 6',7'-dihydroxybergamottin acetonide with related furanocoumarins and coumarins:
Key Findings
Potency Differences: The acetonide derivative exhibits 10–20× greater CYP3A4 inhibitory potency than its parent compound, DHB (IC₅₀: 0.13–0.27 µM vs. 1.58 µM) . This enhancement is attributed to the acetonide group improving metabolic stability and membrane permeability. Bergamottin, another natural furanocoumarin, shows weaker inhibition (IC₅₀: 2.42 µM) and substrate-dependent mechanisms, making it less effective in broad CYP3A4 suppression .
Structural Determinants of Activity: The furanocoumarin core is critical for CYP3A4 inhibition. Coumarins lacking this structure (e.g., marmin acetonide) show negligible activity . Geranyloxy substituents at C5 (bergamottin, DHB) enhance binding to CYP3A4’s active site, while epoxidation (epoxybergamottin) or dihydroxylation (DHB) modifies inhibitory kinetics .
Mechanistic Insights :
- DHB and its acetonide act as substrate-independent inhibitors , blocking CYP3A4 via both reversible binding and irreversible mechanism-based inactivation .
- In contrast, bergamottin’s inhibition is substrate-dependent , limiting its efficacy against diverse CYP3A4 substrates .
Biosynthetic Context :
- DHB, bergamottin, and epoxybergamottin are biosynthetically linked through O-geranylation and oxidation steps in pummelos and grapefruits . The acetonide derivative, however, is exclusively synthetic, highlighting the role of chemical modification in optimizing bioactivity .
Pharmacological and Toxicological Implications
- Drug Interactions: DHB and its acetonide significantly increase the bioavailability of CYP3A4-metabolized drugs (e.g., cyclosporine, statins) by inhibiting intestinal CYP3A4.
- Antimicrobial Activity: DHB exhibits broad-spectrum antibacterial effects (MIC: 1.20–4.80 mg/mL), likely due to its aliphatic chain at C5.
Biological Activity
6',7'-Dihydroxybergamottin acetonide is a furanocoumarin compound predominantly found in citrus fruits such as grapefruit, pomelos, and sour oranges. This compound is recognized for its significant biological activity, particularly in modulating the metabolism of various pharmaceutical drugs through its interaction with cytochrome P450 enzymes, notably CYP3A4. This article reviews the biological activity of this compound, highlighting its mechanisms of action, effects on drug metabolism, and relevant case studies.
- Molecular Formula : C_{22}H_{26}O_5
- Molecular Weight : 398.44 g/mol
- Structure : Characterized by the presence of an acetonide group attached to the furanocoumarin backbone.
This compound primarily functions as an inhibitor of cytochrome P450 3A4 (CYP3A4). This inhibition alters the metabolism of drugs that are substrates for this enzyme, leading to increased bioavailability and potential toxicity. The compound affects both reversible and mechanism-based inhibition of CYP3A4 activity.
Inhibition Profile
- Reversible Inhibition : The compound shows significant reversible inhibition of CYP3A4, with studies indicating a 95% confidence interval for inhibition rates.
- Mechanism-Based Inhibition : Time-dependent loss of CYP3A4 protein has been observed in vitro, establishing the potency of this compound in altering enzyme activity.
Biological Activity and Drug Interactions
The biological activity of this compound has been extensively studied in relation to various drugs. Notably, it enhances the bioavailability of drugs such as felodipine and cyclosporine when consumed with grapefruit juice.
Case Study: Felodipine Interaction
In a clinical study involving healthy volunteers:
- Method : Participants ingested felodipine with grapefruit juice, orange juice-containing serum, or plain orange juice.
- Results : Grapefruit juice significantly increased the area under the plasma concentration-time curve (AUC) for felodipine by 1.9-fold compared to orange juice (P = 0.04) and increased peak concentration by 1.7-fold (P = 0.01) .
Table 1: Summary of Drug Interaction Studies
Clinical Implications
The implications of these interactions are critical in clinical settings where patients may consume grapefruit juice alongside prescribed medications. The potential for increased drug levels can lead to adverse effects or toxicity, necessitating careful management and patient education regarding dietary restrictions.
Q & A
Basic Research Questions
Q. How can researchers confirm the identity and purity of 6',7'-Dihydroxybergamottin acetonide in synthetic preparations?
- Methodological Answer : Use a combination of physical characterization (melting point: 104–106°C , optical rotation: [α]D +12.7 in CHCl3 ) and chromatographic techniques (HPLC or TLC) with authentic standards. Mass spectrometry (MS) and nuclear magnetic resonance (NMR) are critical for structural confirmation, particularly to distinguish between diastereomers or acetonide derivatives .
Q. What are the optimal conditions for synthesizing this compound from its parent compound?
- Methodological Answer : Acetonide formation typically involves reacting dihydroxy precursors with acetone under acidic catalysis (e.g., concentrated H2SO4 or HCl). Selectivity can be achieved by controlling reaction time and temperature, as demonstrated in analogous diacetonide syntheses . Monitor reaction progress via thin-layer chromatography (TLC) to avoid over-acetylation .
Q. What analytical techniques are recommended for structural elucidation of this compound?
- Methodological Answer : High-resolution mass spectrometry (HR-MS) confirms molecular formula (C21H24O6 ). 1D/2D NMR (e.g., COSY, HSQC, HMBC) resolves stereochemistry and acetonide ring conformation. X-ray crystallography may be employed if single crystals are obtainable .
Advanced Research Questions
Q. How does this compound inhibit CYP3A4, and what experimental models validate this mechanism?
- Methodological Answer : Use in vitro CYP3A4 inhibition assays (e.g., fluorogenic substrate metabolism in human liver microsomes) to determine IC50 values. Validate findings with time-dependent inhibition studies and molecular docking simulations to identify binding interactions. Compare results to grapefruit furanocoumarins (e.g., bergamottin) to contextualize potency .
Q. What methodological considerations are critical when designing in vivo studies to assess the pharmacokinetic interactions of this compound?
- Methodological Answer : Prioritize species-specific CYP3A4 expression (e.g., transgenic mice or humanized liver models). Employ factorial design to isolate variables such as dosage, administration route, and co-administered drugs . Use LC-MS/MS for plasma concentration monitoring and metabolite profiling .
Q. How can researchers resolve contradictions in reported bioactivity data for this compound across different studies?
- Methodological Answer : Conduct meta-analyses to identify confounding variables (e.g., solvent polarity in bioassays, purity thresholds). Replicate experiments under standardized conditions (e.g., ISO/IEC 17025 guidelines) and validate using orthogonal assays (e.g., enzymatic vs. cell-based CYP3A4 inhibition) .
Q. What computational strategies are employed to predict the metabolic fate of this compound, and how are these models validated experimentally?
- Methodological Answer : Use quantum mechanical/molecular mechanical (QM/MM) simulations to predict acetonide ring stability under physiological pH. Validate predictions with in vitro hepatic microsomal stability assays and isotopic labeling (e.g., 13C-acetone tracing) to track acetonide hydrolysis .
Q. Key Data from Literature
Property | Value | Source |
---|---|---|
Molecular Formula | C21H24O6 | |
Melting Point | 104–106°C | |
Optical Rotation ([α]D) | +12.7 (c 1.0, CHCl3) | |
Biological Activity | CYP3A4 Inhibition (IC50: <1 µM) | |
Natural Source | Citrus macroptera fruits |
Q. Methodological Best Practices
- Reproducibility : Document solvent purity, instrument calibration, and batch-to-batch variability in synthetic protocols .
- Theoretical Frameworks : Link studies to cytochrome P450 inhibition kinetics or natural product biosynthesis pathways to contextualize findings .
- Ethical Compliance : For human tissue studies, adhere to institutional review board (IRB) protocols for informed consent and data anonymization .
Properties
Molecular Formula |
C24H28O6 |
---|---|
Molecular Weight |
412.5 g/mol |
IUPAC Name |
4-[(E)-3-methyl-5-(2,2,5,5-tetramethyl-1,3-dioxolan-4-yl)pent-2-enoxy]furo[3,2-g]chromen-7-one |
InChI |
InChI=1S/C24H28O6/c1-15(6-8-20-23(2,3)30-24(4,5)29-20)10-12-27-22-16-7-9-21(25)28-19(16)14-18-17(22)11-13-26-18/h7,9-11,13-14,20H,6,8,12H2,1-5H3/b15-10+ |
InChI Key |
DLFOGGFNAICJAO-XNTDXEJSSA-N |
Isomeric SMILES |
C/C(=C\COC1=C2C=CC(=O)OC2=CC3=C1C=CO3)/CCC4C(OC(O4)(C)C)(C)C |
Canonical SMILES |
CC(=CCOC1=C2C=CC(=O)OC2=CC3=C1C=CO3)CCC4C(OC(O4)(C)C)(C)C |
Origin of Product |
United States |
Disclaimer and Information on In-Vitro Research Products
Please be aware that all articles and product information presented on BenchChem are intended solely for informational purposes. The products available for purchase on BenchChem are specifically designed for in-vitro studies, which are conducted outside of living organisms. In-vitro studies, derived from the Latin term "in glass," involve experiments performed in controlled laboratory settings using cells or tissues. It is important to note that these products are not categorized as medicines or drugs, and they have not received approval from the FDA for the prevention, treatment, or cure of any medical condition, ailment, or disease. We must emphasize that any form of bodily introduction of these products into humans or animals is strictly prohibited by law. It is essential to adhere to these guidelines to ensure compliance with legal and ethical standards in research and experimentation.