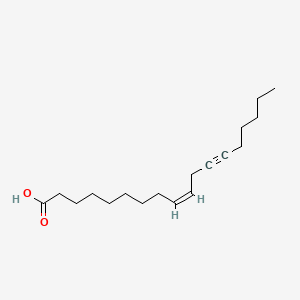
Crepenynic acid
Overview
Description
It is predominantly found in the seed oils of certain plants, particularly those belonging to the Asteraceae family, such as Crepis rubra and Atractylodes species . This compound is characterized by the presence of a triple bond (acetylenic bond) at the twelfth carbon of its 18-carbon chain, which grants it unique chemical properties .
Mechanism of Action
Crepenynic acid, also known as (Z)-octadec-9-en-12-ynoic acid, is a specialized fatty acid known for its unique acetylenic bond located at the ninth carbon of its 18-carbon chain . This compound has been the subject of various research contexts due to its distinctive chemical properties .
Target of Action
This compound’s primary targets are the biosynthetic enzymes involved in the formation of alkyne . These enzymes play a crucial role in the conversion of linoleic acid to this compound, which is the first step of falcarindiol biosynthesis in tomato .
Mode of Action
This compound interacts with its targets, the acetylenases, which are encoded by ACET1a and ACET1b . These identical acetylenases catalyze the conversion of linoleic acid to this compound . This interaction results in the formation of a triple bond at the Δ12 position of linoleic acid, forming this compound .
Biochemical Pathways
This compound is involved in the biosynthesis of alkyne-containing natural products . It is derived biosynthetically from linoleic acid and is itself the precursor of a substantial number of acetylenic metabolites with diverse biological functions in plants . The conversion of linoleic acid to this compound is the first step of falcarindiol biosynthesis in tomato .
Pharmacokinetics
It is known that this compound is a major component of certain seed oils of higher plants . This suggests that the compound’s bioavailability may be influenced by its presence in these oils.
Result of Action
The result of this compound’s action is the production of a variety of acetylenic metabolites with diverse biological functions in plants . These metabolites are often used as defense compounds and have shown potential pharmaceutical properties, such as antibacterial and anti-cancer agents .
Action Environment
This compound’s action, efficacy, and stability can be influenced by various environmental factors. For instance, it is chemically unstable and highly reactive, especially in the presence of oxygen . Furthermore, its production is often concentrated in specific tissues, such as the seeds of certain plants , suggesting that its action may be influenced by the specific environment within these tissues.
Biochemical Analysis
Biochemical Properties
Crepenynic acid plays a crucial role in biochemical reactions, particularly in the biosynthesis of polyacetylenic compounds. The conversion of linoleic acid to this compound is catalyzed by acetylenase enzymes, such as ACET1a and ACET1b . These enzymes facilitate the insertion of a triple bond at the Δ12 position of linoleic acid, forming this compound . Additionally, this compound interacts with various biomolecules, including cytochrome b5, which provides the necessary electrons for the desaturation process . The presence of this compound in plant tissues is associated with its role in plant defense mechanisms, potentially deterring pests due to its reactive acetylenic bond .
Cellular Effects
This compound exerts several effects on various types of cells and cellular processes. In plants, it is involved in the biosynthesis of falcarindiol, a dietary metabolite with antifungal activity and cytotoxicity against several human cancer cell lines . The presence of this compound in plant tissues has been linked to its role in plant defense, where it may interfere with animal and insect metabolism . Furthermore, this compound has been shown to influence cell signaling pathways and gene expression, contributing to its biological activity .
Molecular Mechanism
The molecular mechanism of this compound involves its interaction with specific enzymes and biomolecules. The acetylenase enzymes ACET1a and ACET1b catalyze the conversion of linoleic acid to this compound through an initial hydrogen atom abstraction at the C12 position of the linoleoyl substrate . This reaction is followed by the insertion of a triple bond, resulting in the formation of this compound . The unique acetylenic bond in this compound grants it distinctive chemical properties, making it an intriguing subject in various research contexts .
Temporal Effects in Laboratory Settings
In laboratory settings, the effects of this compound have been observed to change over time. The stability and degradation of this compound are influenced by various factors, including the presence of specific enzymes and cofactors . In developing seeds of Crepis alpina, this compound accumulates at high levels, while other tissues contain none . This tissue-specific accumulation is attributed to the co-expression of acetylenase and other desaturases, which may contribute to the stability and long-term effects of this compound in plant tissues .
Dosage Effects in Animal Models
The effects of this compound vary with different dosages in animal models. While specific studies on the dosage effects of this compound in animal models are limited, it is known that the compound’s biological activity can be influenced by its concentration . High doses of this compound may exhibit toxic or adverse effects, while lower doses may have beneficial effects on cellular function and metabolism
Metabolic Pathways
This compound is involved in several metabolic pathways, including the biosynthesis of polyacetylenic compounds. The conversion of linoleic acid to this compound is catalyzed by acetylenase enzymes, which introduce a triple bond at the Δ12 position of linoleic acid . This reaction is the first step in the biosynthesis of falcarindiol, a polyacetylene with antifungal and cytotoxic properties .
Transport and Distribution
The transport and distribution of this compound within cells and tissues are influenced by its interactions with specific transporters and binding proteins. In the seed oils of Atractylodes lancea and A. macrocephala, this compound is predominantly found in the triacylglycerol fraction, with a contrasting distribution observed in different species . The localization and accumulation of this compound in plant tissues are likely regulated by its synthesis and assembly into triacylglycerols
Subcellular Localization
The subcellular localization of this compound is primarily within the endoplasmic reticulum, where the acetylenase enzymes responsible for its biosynthesis are located . The endoplasmic reticulum provides the necessary environment for the desaturation and acetylenation reactions that produce this compound . Additionally, the subcellular localization of this compound may be influenced by targeting signals and post-translational modifications that direct it to specific compartments or organelles . Understanding the subcellular localization of this compound is crucial for elucidating its role in cellular metabolism and function.
Preparation Methods
Synthetic Routes and Reaction Conditions: Crepenynic acid is biosynthesized in plants through the introduction of a triple bond into an existing fatty acid. The precursor for this biosynthesis is typically linoleic acid (cis-9,12-octadecadienoic acid). The enzyme responsible for this transformation is an acetylenase, which catalyzes the conversion of linoleic acid to this compound .
Industrial Production Methods: Industrial production of this compound is not widely established due to its natural occurrence in specific plant species. extraction from seed oils of plants like Crepis rubra and Atractylodes lancea is a common method. The extraction process involves the use of solvents and subsequent purification steps to isolate the fatty acid .
Chemical Reactions Analysis
Types of Reactions: Crepenynic acid undergoes various chemical reactions, including:
Reduction: Reduction of the triple bond can yield saturated or partially saturated fatty acids.
Substitution: The acetylenic bond can participate in substitution reactions, forming various substituted derivatives.
Common Reagents and Conditions:
Oxidation: Common oxidizing agents include potassium permanganate and osmium tetroxide.
Reduction: Hydrogenation using palladium or platinum catalysts.
Substitution: Halogenation using bromine or chlorine under controlled conditions.
Major Products:
Oxidation: Epoxides and hydroxylated derivatives.
Reduction: Stearic acid and oleic acid derivatives.
Substitution: Halogenated fatty acids.
Scientific Research Applications
Crepenynic acid has several scientific research applications:
Chemistry: Used as a probe to study enzymatic pathways involved in the biosynthesis of polyacetylenic compounds.
Medicine: Explored for its antibacterial and anticancer properties.
Comparison with Similar Compounds
- Tariric acid (octadec-6-ynoic acid)
- Stearolic acid (octadec-9-ynoic acid)
- Sterculynic acid (cis-9,10-methylene-9-octadecen-17-ynoic acid)
Comparison: Crepenynic acid is unique due to its specific acetylenic bond position at the twelfth carbon, which is distinct from other acetylenic fatty acids like tariric and stearolic acids. This unique positioning grants this compound different chemical reactivity and biological functions . Additionally, this compound is a precursor for a substantial number of polyacetylenic metabolites, making it a critical compound in the biosynthesis of various bioactive molecules .
Properties
IUPAC Name |
(Z)-octadec-9-en-12-ynoic acid | |
---|---|---|
Source | PubChem | |
URL | https://pubchem.ncbi.nlm.nih.gov | |
Description | Data deposited in or computed by PubChem | |
InChI |
InChI=1S/C18H30O2/c1-2-3-4-5-6-7-8-9-10-11-12-13-14-15-16-17-18(19)20/h9-10H,2-5,8,11-17H2,1H3,(H,19,20)/b10-9- | |
Source | PubChem | |
URL | https://pubchem.ncbi.nlm.nih.gov | |
Description | Data deposited in or computed by PubChem | |
InChI Key |
SAOSKFBYQJLQOS-KTKRTIGZSA-N | |
Source | PubChem | |
URL | https://pubchem.ncbi.nlm.nih.gov | |
Description | Data deposited in or computed by PubChem | |
Canonical SMILES |
CCCCCC#CCC=CCCCCCCCC(=O)O | |
Source | PubChem | |
URL | https://pubchem.ncbi.nlm.nih.gov | |
Description | Data deposited in or computed by PubChem | |
Isomeric SMILES |
CCCCCC#CC/C=C\CCCCCCCC(=O)O | |
Source | PubChem | |
URL | https://pubchem.ncbi.nlm.nih.gov | |
Description | Data deposited in or computed by PubChem | |
Molecular Formula |
C18H30O2 | |
Source | PubChem | |
URL | https://pubchem.ncbi.nlm.nih.gov | |
Description | Data deposited in or computed by PubChem | |
DSSTOX Substance ID |
DTXSID201305279 | |
Record name | (9Z)-9-Octadecen-12-ynoic acid | |
Source | EPA DSSTox | |
URL | https://comptox.epa.gov/dashboard/DTXSID201305279 | |
Description | DSSTox provides a high quality public chemistry resource for supporting improved predictive toxicology. | |
Molecular Weight |
278.4 g/mol | |
Source | PubChem | |
URL | https://pubchem.ncbi.nlm.nih.gov | |
Description | Data deposited in or computed by PubChem | |
CAS No. |
2277-31-8 | |
Record name | (9Z)-9-Octadecen-12-ynoic acid | |
Source | CAS Common Chemistry | |
URL | https://commonchemistry.cas.org/detail?cas_rn=2277-31-8 | |
Description | CAS Common Chemistry is an open community resource for accessing chemical information. Nearly 500,000 chemical substances from CAS REGISTRY cover areas of community interest, including common and frequently regulated chemicals, and those relevant to high school and undergraduate chemistry classes. This chemical information, curated by our expert scientists, is provided in alignment with our mission as a division of the American Chemical Society. | |
Explanation | The data from CAS Common Chemistry is provided under a CC-BY-NC 4.0 license, unless otherwise stated. | |
Record name | (9Z)-9-Octadecen-12-ynoic acid | |
Source | EPA DSSTox | |
URL | https://comptox.epa.gov/dashboard/DTXSID201305279 | |
Description | DSSTox provides a high quality public chemistry resource for supporting improved predictive toxicology. | |
Retrosynthesis Analysis
AI-Powered Synthesis Planning: Our tool employs the Template_relevance Pistachio, Template_relevance Bkms_metabolic, Template_relevance Pistachio_ringbreaker, Template_relevance Reaxys, Template_relevance Reaxys_biocatalysis model, leveraging a vast database of chemical reactions to predict feasible synthetic routes.
One-Step Synthesis Focus: Specifically designed for one-step synthesis, it provides concise and direct routes for your target compounds, streamlining the synthesis process.
Accurate Predictions: Utilizing the extensive PISTACHIO, BKMS_METABOLIC, PISTACHIO_RINGBREAKER, REAXYS, REAXYS_BIOCATALYSIS database, our tool offers high-accuracy predictions, reflecting the latest in chemical research and data.
Strategy Settings
Precursor scoring | Relevance Heuristic |
---|---|
Min. plausibility | 0.01 |
Model | Template_relevance |
Template Set | Pistachio/Bkms_metabolic/Pistachio_ringbreaker/Reaxys/Reaxys_biocatalysis |
Top-N result to add to graph | 6 |
Feasible Synthetic Routes
Q1: What is the natural source of crepenynic acid?
A1: this compound is found in the seed oils of certain plants, particularly those belonging to the Asteraceae family. A significant source is Crepis alpina, from which the compound derives its name. [, , ]
Q2: How is this compound biosynthesized in plants?
A2: this compound biosynthesis involves the desaturation of linoleic acid. A specific enzyme, Δ12 acetylenase (a variant of FAD2 desaturase), catalyzes the introduction of a triple bond at the Δ12 position of linoleic acid, leading to the formation of this compound. [, , , , ]
Q3: Are there other plant species besides Crepis alpina that are known to contain this compound?
A3: Yes, besides Crepis alpina, this compound has been identified in other plant species, including Afzelia spp., Saussurea candicans, Atractylodes lancea, and Atractylodes macrocephala. [, , ]
Q4: What is the molecular formula and molecular weight of this compound?
A4: The molecular formula of this compound is C18H30O2, and its molecular weight is 278.43 g/mol. [, , ]
Q5: What spectroscopic techniques have been used to characterize this compound?
A5: Several spectroscopic techniques have been employed for the characterization of this compound and its derivatives, including gas chromatography-mass spectrometry (GC-MS), infrared (IR) spectroscopy, nuclear magnetic resonance (NMR) spectroscopy, and silver ion thin-layer chromatography. [, , , , ]
Q6: How does the presence of both a double bond and a triple bond in this compound influence its properties?
A6: The unique structure of this compound with both a double bond and a triple bond impacts its reactivity and potential applications. The triple bond, for instance, makes it susceptible to oxidation, while the presence of both functionalities makes it suitable for cross-linking reactions in polymer chemistry. [, ]
Q7: What is known about the metabolic fate of this compound in animal models?
A7: Studies in rats have shown that dietary this compound, when administered as a naturally occurring triglyceride, is not significantly incorporated into brain, liver, or depot fat. A small amount is metabolized and excreted in the urine as 4-decynedioic acid. []
Q8: Does this compound interact with enzymes involved in fatty acid metabolism?
A8: Yes, this compound has been investigated as a potential inhibitor of essential fatty acid metabolism. [] Additionally, it has been shown to interact with lipoxygenase, an enzyme involved in the oxidation of polyunsaturated fatty acids. []
Q9: How does this compound affect lipoxygenase activity?
A9: this compound acts as a mechanism-based inhibitor of lipoxygenase. During its enzymatic conversion, this compound generates hydroperoxide products that irreversibly inactivate the enzyme. The mechanism involves the oxidation of Fe(II)-lipoxygenase to its Fe(III) state and the generation of reactive radical species. []
Q10: What are the synthetic routes for obtaining this compound?
A10: Several methods have been developed for the synthesis of this compound, including:
- A multi-step synthesis utilizing non-3-yn-1-yl-triphenylphosphonium bromide and a C9 aldehyde-ester. []
- A method involving 1,4-diene and 1,4-enyne synthesis via dichloronorcarenol cleavage. [, ]
- Partial synthesis starting from naturally occurring this compound, leading to the production of racemic helenynolic acid. [, ]
Q11: What are the potential applications of this compound and its derivatives?
A11: Due to its unique structure and reactivity, this compound and its derivatives hold potential for various applications, including:
- Monomers in coating applications: this compound's alkyne functionality allows its use in crosslinking reactions to form polymers with desired properties. []
- Potential pharmaceuticals: Research suggests potential therapeutic applications for this compound and related compounds, though further investigation is needed. []
- Chemical probes: The specific interaction of this compound with enzymes like lipoxygenase makes it a valuable tool for studying enzyme mechanisms and developing potential inhibitors. []
Q12: What analytical methods are used to study this compound?
A12: Analytical techniques used for studying this compound include GC-MS for identification and quantification, as well as high-performance liquid chromatography (HPLC) for separating and analyzing its triacylglycerols. [, ]
Disclaimer and Information on In-Vitro Research Products
Please be aware that all articles and product information presented on BenchChem are intended solely for informational purposes. The products available for purchase on BenchChem are specifically designed for in-vitro studies, which are conducted outside of living organisms. In-vitro studies, derived from the Latin term "in glass," involve experiments performed in controlled laboratory settings using cells or tissues. It is important to note that these products are not categorized as medicines or drugs, and they have not received approval from the FDA for the prevention, treatment, or cure of any medical condition, ailment, or disease. We must emphasize that any form of bodily introduction of these products into humans or animals is strictly prohibited by law. It is essential to adhere to these guidelines to ensure compliance with legal and ethical standards in research and experimentation.