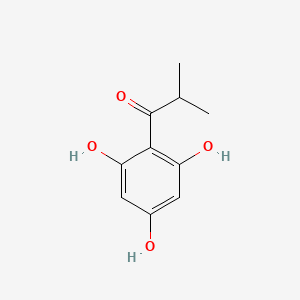
Phlorisobutyrophenone
Overview
Description
Phloroisobutyrophenone is a chemical compound known for its role as an intermediate in the biosynthesis of various bioactive compounds.
Mechanism of Action
Target of Action
Phlorisobutyrophenone primarily targets Type III polyketide synthases (PKSs) . These enzymes contribute to the synthesis of many economically important natural products . More specifically, this compound is synthesized by the enzyme phloroisovalerophenone synthase , which also acts on isobutyryl-CoA as a substrate .
Mode of Action
This compound is synthesized by the action of phloroisovalerophenone synthase . This enzyme catalyzes the transfer of a dimethylallyl moiety to phloroglucinol derivatives, leading to the formation of this compound .
Biochemical Pathways
The biosynthesis of this compound involves a pathway of isovaleryl-CoA via hydroxy-3-methylglutaryl CoA (HMG-CoA) , followed by dehydration, decarboxylation, and reduction in E. coli . The type III PKSs valerophenone synthase or chalcone synthase from plants are then introduced to produce this compound .
Pharmacokinetics
coli from glucose , suggesting that its bioavailability may be influenced by factors such as glucose availability and the metabolic state of the organism.
Result of Action
The products of the action of phloroisovalerophenone synthase, including this compound, are intermediates in the biosynthesis of the bitter acids in hops (Humulus lupulus) and glucosides in strawberry (Fragaria X ananassa) . These compounds play important roles in human nutrition and health, and have recently expanded their roles as platform chemicals .
Action Environment
The action of this compound synthase and the resulting production of this compound can be influenced by environmental factors such as the availability of substrates (e.g., glucose) and the metabolic state of the organism (e.g., E. coli)
Biochemical Analysis
Biochemical Properties
Phlorisobutyrophenone plays a significant role in biochemical reactions, particularly in the prenylation process. Prenylation is a post-translational modification where prenyl groups are added to proteins or other molecules, influencing their function and localization. This compound interacts with enzymes such as prenyltransferases, which catalyze the transfer of prenyl groups to aromatic compounds. For instance, the enzyme HlPT-1 catalyzes the transfer of a dimethylallyl moiety to this compound, leading to the formation of humulone and lupulone derivatives . These interactions are crucial for the biosynthesis of various secondary metabolites that have pharmacological and industrial applications.
Cellular Effects
This compound affects various cellular processes, including cell signaling pathways, gene expression, and cellular metabolism. In microbial cells, such as Escherichia coli, this compound is involved in the biosynthesis of key intermediates like humulone, which contributes to the characteristic bitter taste of beer . This compound influences cell function by modulating the activity of enzymes involved in its biosynthetic pathway, thereby affecting the overall metabolic flux and production of secondary metabolites.
Molecular Mechanism
At the molecular level, this compound exerts its effects through specific binding interactions with biomolecules. It acts as a substrate for prenyltransferases, which facilitate the addition of prenyl groups to its aromatic ring. This modification enhances the compound’s lipophilicity and binding affinity to target proteins, thereby influencing its biological activity . Additionally, this compound can modulate enzyme activity, either by inhibition or activation, depending on the specific biochemical context.
Temporal Effects in Laboratory Settings
In laboratory settings, the effects of this compound can change over time due to its stability and degradation properties. Studies have shown that this compound remains stable under specific conditions, but its activity can be influenced by factors such as temperature and pH . Long-term effects on cellular function have been observed in in vitro studies, where prolonged exposure to this compound can lead to changes in metabolic pathways and gene expression profiles.
Dosage Effects in Animal Models
The effects of this compound vary with different dosages in animal models. At low doses, it may exhibit beneficial effects by modulating metabolic pathways and enhancing the production of secondary metabolites. At high doses, this compound can exhibit toxic or adverse effects, such as enzyme inhibition and disruption of cellular homeostasis . These threshold effects highlight the importance of dosage optimization in experimental studies.
Metabolic Pathways
This compound is involved in several metabolic pathways, particularly those related to the biosynthesis of prenylated compounds. It interacts with enzymes such as valerophenone synthase and chalcone synthase, which catalyze the formation of its acylphloroglucinol core . These interactions influence the overall metabolic flux and levels of metabolites, contributing to the production of biologically active compounds like humulone and lupulone.
Transport and Distribution
Within cells and tissues, this compound is transported and distributed through specific transporters and binding proteins. Its localization and accumulation are influenced by its interactions with these transporters, which facilitate its movement across cellular compartments . The distribution of this compound is also affected by its lipophilicity, which allows it to integrate into lipid membranes and interact with membrane-bound proteins.
Subcellular Localization
This compound exhibits specific subcellular localization patterns, which are crucial for its activity and function. It is often localized in chloroplasts and other organelles involved in secondary metabolite biosynthesis . This localization is directed by targeting signals and post-translational modifications that ensure its proper compartmentalization within the cell. The subcellular distribution of this compound influences its interactions with enzymes and other biomolecules, thereby modulating its biochemical effects.
Preparation Methods
Synthetic Routes and Reaction Conditions
Phloroisobutyrophenone can be synthesized through the Claisen condensation of isobutyryl-CoA with phloroglucinol. This reaction is catalyzed by enzymes such as valerophenone synthase or chalcone synthase . The reaction conditions typically involve the use of a suitable solvent and a controlled temperature to ensure the proper formation of the desired product.
Industrial Production Methods
In industrial settings, phloroisobutyrophenone can be produced using microbial fermentation processes. For example, Escherichia coli can be genetically engineered to express the necessary enzymes for the biosynthesis of phloroisobutyrophenone from glucose . This method offers a sustainable and scalable approach to producing the compound.
Chemical Reactions Analysis
Types of Reactions
Phloroisobutyrophenone undergoes various chemical reactions, including:
Oxidation: It can be oxidized to form corresponding quinones.
Reduction: Reduction reactions can convert it into alcohol derivatives.
Substitution: It can participate in substitution reactions, where functional groups are replaced by others.
Common Reagents and Conditions
Common reagents used in these reactions include oxidizing agents like potassium permanganate for oxidation, reducing agents such as sodium borohydride for reduction, and various nucleophiles for substitution reactions. The conditions for these reactions vary depending on the desired product but generally involve controlled temperatures and specific solvents .
Major Products Formed
The major products formed from these reactions include quinones, alcohol derivatives, and substituted phloroglucinol compounds. These products have diverse applications in different fields, including pharmaceuticals and materials science .
Scientific Research Applications
Chemistry: It serves as an intermediate in the synthesis of complex organic molecules.
Biology: It is used in the study of enzyme-catalyzed reactions and metabolic pathways.
Industry: It is used in the production of specialty chemicals and materials.
Comparison with Similar Compounds
Phloroisobutyrophenone is similar to other acylphloroglucinol derivatives such as phlorisovalerophenone and phloroglucinol. it is unique in its specific structure and the types of reactions it undergoes. Unlike phlorisovalerophenone, which uses isovaleryl-CoA, phloroisobutyrophenone uses isobutyryl-CoA as a substrate. This difference in substrate specificity highlights its uniqueness and potential for diverse applications.
List of Similar Compounds
- Phlorisovalerophenone
- Phloroglucinol
- Hyperforin (a derivative of phloroglucinol)
Properties
IUPAC Name |
2-methyl-1-(2,4,6-trihydroxyphenyl)propan-1-one | |
---|---|---|
Source | PubChem | |
URL | https://pubchem.ncbi.nlm.nih.gov | |
Description | Data deposited in or computed by PubChem | |
InChI |
InChI=1S/C10H12O4/c1-5(2)10(14)9-7(12)3-6(11)4-8(9)13/h3-5,11-13H,1-2H3 | |
Source | PubChem | |
URL | https://pubchem.ncbi.nlm.nih.gov | |
Description | Data deposited in or computed by PubChem | |
InChI Key |
BNEBXEZRBLYBCZ-UHFFFAOYSA-N | |
Source | PubChem | |
URL | https://pubchem.ncbi.nlm.nih.gov | |
Description | Data deposited in or computed by PubChem | |
Canonical SMILES |
CC(C)C(=O)C1=C(C=C(C=C1O)O)O | |
Source | PubChem | |
URL | https://pubchem.ncbi.nlm.nih.gov | |
Description | Data deposited in or computed by PubChem | |
Molecular Formula |
C10H12O4 | |
Source | PubChem | |
URL | https://pubchem.ncbi.nlm.nih.gov | |
Description | Data deposited in or computed by PubChem | |
DSSTOX Substance ID |
DTXSID60416108 | |
Record name | Phlorisobutyrophenone | |
Source | EPA DSSTox | |
URL | https://comptox.epa.gov/dashboard/DTXSID60416108 | |
Description | DSSTox provides a high quality public chemistry resource for supporting improved predictive toxicology. | |
Molecular Weight |
196.20 g/mol | |
Source | PubChem | |
URL | https://pubchem.ncbi.nlm.nih.gov | |
Description | Data deposited in or computed by PubChem | |
CAS No. |
35458-21-0 | |
Record name | Phlorisobutyrophenone | |
Source | EPA DSSTox | |
URL | https://comptox.epa.gov/dashboard/DTXSID60416108 | |
Description | DSSTox provides a high quality public chemistry resource for supporting improved predictive toxicology. | |
Synthesis routes and methods
Procedure details
Retrosynthesis Analysis
AI-Powered Synthesis Planning: Our tool employs the Template_relevance Pistachio, Template_relevance Bkms_metabolic, Template_relevance Pistachio_ringbreaker, Template_relevance Reaxys, Template_relevance Reaxys_biocatalysis model, leveraging a vast database of chemical reactions to predict feasible synthetic routes.
One-Step Synthesis Focus: Specifically designed for one-step synthesis, it provides concise and direct routes for your target compounds, streamlining the synthesis process.
Accurate Predictions: Utilizing the extensive PISTACHIO, BKMS_METABOLIC, PISTACHIO_RINGBREAKER, REAXYS, REAXYS_BIOCATALYSIS database, our tool offers high-accuracy predictions, reflecting the latest in chemical research and data.
Strategy Settings
Precursor scoring | Relevance Heuristic |
---|---|
Min. plausibility | 0.01 |
Model | Template_relevance |
Template Set | Pistachio/Bkms_metabolic/Pistachio_ringbreaker/Reaxys/Reaxys_biocatalysis |
Top-N result to add to graph | 6 |
Feasible Synthetic Routes
Q1: What is phlorisobutyrophenone and where is it found?
A1: this compound is an acylphloroglucinol, a class of natural products known for their diverse biological activities. It serves as a key intermediate in the biosynthesis of more complex acylphloroglucinols, including those found in the genus Hypericum. [, , , ] Hypericum species, particularly Hypericum perforatum (St. John's Wort), are well-known for their medicinal properties. []
Q2: How is this compound biosynthesized?
A2: this compound is synthesized from isobutyryl-CoA and three molecules of malonyl-CoA through the action of enzymes known as phlorisovalerophenone synthases (VPS) or chalcone synthases (CHS) exhibiting valerophenone synthase activity. These enzymes belong to the family of plant polyketide synthases. [, , ] Studies have shown that certain CHS enzymes in strawberry (Fragaria x ananassa) possess dual functionality, acting as both CHS and phlorisovalerophenone synthases, contributing to the production of this compound. []
Q3: Can you describe the enzymatic synthesis of this compound in more detail?
A3: this compound synthase (VPS), a specific type of polyketide synthase, has been isolated and characterized from hop (Humulus lupulus L.) cones. [] This enzyme catalyzes the condensation of isobutyryl-CoA with three molecules of malonyl-CoA to form this compound, a key precursor for the bitter acids found in hops. []
Q4: What are the potential applications of this compound?
A4: While this compound itself might not possess potent biological activity, it serves as a crucial precursor for synthesizing various bioactive compounds. For instance, in Hypericum sampsonii, this compound is prenylated by specific prenyltransferases to yield more complex and biologically active polycyclic polyprenylated acylphloroglucinols (PPAPs). [] These PPAPs have shown promising cytotoxic and anti-inflammatory activities. []
Q5: Are there any analytical techniques available to identify and quantify this compound in plant extracts?
A5: Yes, this compound can be identified and quantified using techniques like liquid chromatography/electrospray ionization-mass spectrometry (LC/ESI-MS) and high-performance liquid chromatography coupled with ultraviolet detection (HPLC-UV). [] These techniques are valuable tools for studying the presence and variation of this compound and other related acylphloroglucinols in different plant species and tissues. []
Q6: What are the future directions for research on this compound?
A6: Future research on this compound could focus on:
Disclaimer and Information on In-Vitro Research Products
Please be aware that all articles and product information presented on BenchChem are intended solely for informational purposes. The products available for purchase on BenchChem are specifically designed for in-vitro studies, which are conducted outside of living organisms. In-vitro studies, derived from the Latin term "in glass," involve experiments performed in controlled laboratory settings using cells or tissues. It is important to note that these products are not categorized as medicines or drugs, and they have not received approval from the FDA for the prevention, treatment, or cure of any medical condition, ailment, or disease. We must emphasize that any form of bodily introduction of these products into humans or animals is strictly prohibited by law. It is essential to adhere to these guidelines to ensure compliance with legal and ethical standards in research and experimentation.