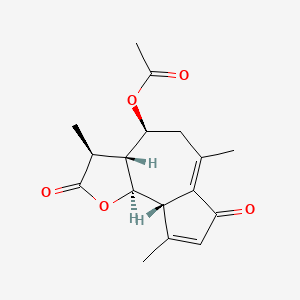
Matricarin
- Click on QUICK INQUIRY to receive a quote from our team of experts.
- With the quality product at a COMPETITIVE price, you can focus more on your research.
Overview
Description
Preparation Methods
Synthetic Routes and Reaction Conditions
Matricarin can be synthesized through several chemical routes. One common method involves the extraction of chamomile flowers, followed by purification using chromatographic techniques . The reaction conditions typically involve the use of organic solvents and controlled temperatures to ensure the stability of the compound .
Industrial Production Methods
Industrial production of this compound often involves large-scale extraction from chamomile flowers. The flowers are dried using methods such as sun drying, shade drying, or using a climate chamber . The dried flowers are then subjected to solvent extraction, followed by purification processes like distillation and chromatography to isolate this compound .
Chemical Reactions Analysis
Types of Reactions
Matricarin undergoes various chemical reactions, including:
Oxidation: This compound can be oxidized to form different derivatives, which may have distinct biological activities.
Reduction: Reduction reactions can modify the lactone ring, altering the compound’s properties.
Common Reagents and Conditions
Common reagents used in these reactions include oxidizing agents like potassium permanganate and reducing agents like sodium borohydride . The reactions are typically carried out under controlled temperatures and pH conditions to ensure the desired transformations .
Major Products
Scientific Research Applications
Mechanism of Action
Matricarin exerts its effects through several molecular targets and pathways. It is known to inhibit pro-inflammatory biomarkers in macrophages, similar to non-steroidal anti-inflammatory drugs (NSAIDs) . Additionally, it affects volume-activated potassium and calcium channels, influencing cellular responses under osmotic stress .
Comparison with Similar Compounds
Matricarin is unique among sesquiterpene lactones due to its specific structure and biological activities. Similar compounds include:
Austricin: Another sesquiterpene lactone with similar anti-inflammatory properties.
Canin: Known for its antimicrobial activity.
Achillin: Exhibits both anti-inflammatory and antimicrobial properties.
Leucodin: A sesquiterpene lactone with antioxidant properties.
Desacetylthis compound: A derivative of this compound with distinct biological activities.
This compound stands out due to its unique combination of anti-inflammatory, antioxidant, and antimicrobial properties, making it a versatile compound for various applications .
Properties
CAS No. |
5989-43-5 |
---|---|
Molecular Formula |
C17H20O5 |
Molecular Weight |
304.34 g/mol |
IUPAC Name |
[(3S,3aR,4S,9aS,9bR)-3,6,9-trimethyl-2,7-dioxo-3,3a,4,5,9a,9b-hexahydroazuleno[4,5-b]furan-4-yl] acetate |
InChI |
InChI=1S/C17H20O5/c1-7-5-11(19)13-8(2)6-12(21-10(4)18)15-9(3)17(20)22-16(15)14(7)13/h5,9,12,14-16H,6H2,1-4H3/t9-,12-,14-,15+,16+/m0/s1 |
InChI Key |
QONYNSMAVSRIRD-UPQAZBFISA-N |
Isomeric SMILES |
C[C@H]1[C@@H]2[C@H](CC(=C3[C@@H]([C@H]2OC1=O)C(=CC3=O)C)C)OC(=O)C |
Canonical SMILES |
CC1C2C(CC(=C3C(C2OC1=O)C(=CC3=O)C)C)OC(=O)C |
melting_point |
193 - 195 °C |
physical_description |
Solid |
Origin of Product |
United States |
Disclaimer and Information on In-Vitro Research Products
Please be aware that all articles and product information presented on BenchChem are intended solely for informational purposes. The products available for purchase on BenchChem are specifically designed for in-vitro studies, which are conducted outside of living organisms. In-vitro studies, derived from the Latin term "in glass," involve experiments performed in controlled laboratory settings using cells or tissues. It is important to note that these products are not categorized as medicines or drugs, and they have not received approval from the FDA for the prevention, treatment, or cure of any medical condition, ailment, or disease. We must emphasize that any form of bodily introduction of these products into humans or animals is strictly prohibited by law. It is essential to adhere to these guidelines to ensure compliance with legal and ethical standards in research and experimentation.