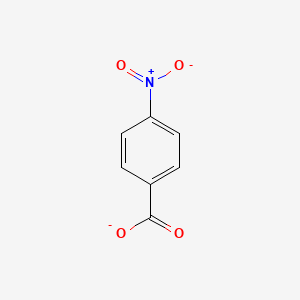
4-Nitrobenzoate
Overview
Description
4-Nitrobenzoate is an organic compound with the molecular formula C7H5NO4. It is a derivative of benzoic acid, where a nitro group (-NO2) is substituted at the para position of the benzene ring. This compound is a pale yellow crystalline solid and is known for its applications in the synthesis of dyes, pharmaceuticals, and as an intermediate in various chemical reactions .
Preparation Methods
Synthetic Routes and Reaction Conditions: 4-Nitrobenzoate can be synthesized through the nitration of benzoic acid. The nitration process involves the reaction of benzoic acid with a mixture of concentrated sulfuric acid and nitric acid. The reaction is typically carried out at a temperature range of 50-60°C to ensure the selective formation of the para-nitro product .
Industrial Production Methods: In industrial settings, this compound is often produced by the oxidation of 4-nitrotoluene. This process involves the use of oxidizing agents such as potassium permanganate or chromic acid. The reaction is conducted under controlled conditions to achieve high yields and purity of the product .
Chemical Reactions Analysis
Types of Reactions: 4-Nitrobenzoate undergoes various chemical reactions, including:
Reduction: The nitro group can be reduced to an amino group using reducing agents like hydrogen gas in the presence of a palladium catalyst or sodium borohydride.
Common Reagents and Conditions:
Reduction: Hydrogen gas with palladium catalyst, sodium borohydride.
Substitution: Hydroxide ions, amines.
Major Products Formed:
Reduction: 4-Aminobenzoate.
Substitution: Various substituted benzoates depending on the nucleophile used.
Scientific Research Applications
4-Nitrobenzoate has a wide range of applications in scientific research:
Chemistry: It is used as an intermediate in the synthesis of various organic compounds, including dyes and pharmaceuticals.
Biology: It serves as a substrate in enzymatic studies to understand the mechanisms of nitroreductases.
Medicine: It is a precursor in the synthesis of local anesthetics such as benzocaine and procaine.
Industry: It is used in the production of polymers and resins.
Mechanism of Action
The mechanism of action of 4-Nitrobenzoate primarily involves its reduction to 4-aminobenzoate. This reduction is catalyzed by nitroreductase enzymes, which facilitate the transfer of electrons to the nitro group, converting it to an amino group. This transformation is crucial in the biosynthesis of various biologically active compounds .
Comparison with Similar Compounds
4-Aminobenzoate: The reduced form of 4-Nitrobenzoate, used in the synthesis of folic acid.
3-Nitrobenzoate: An isomer with the nitro group at the meta position, used in different chemical reactions.
2-Nitrobenzoate: An isomer with the nitro group at the ortho position, also used in various synthetic applications.
Uniqueness: this compound is unique due to its specific positioning of the nitro group, which makes it highly reactive in nucleophilic aromatic substitution reactions. This reactivity is leveraged in the synthesis of a wide range of chemical products, making it a valuable compound in both research and industrial applications .
Properties
CAS No. |
7227-54-5 |
---|---|
Molecular Formula |
C7H4NO4- |
Molecular Weight |
166.11 g/mol |
IUPAC Name |
4-nitrobenzoate |
InChI |
InChI=1S/C7H5NO4/c9-7(10)5-1-3-6(4-2-5)8(11)12/h1-4H,(H,9,10)/p-1 |
InChI Key |
OTLNPYWUJOZPPA-UHFFFAOYSA-M |
SMILES |
C1=CC(=CC=C1C(=O)[O-])[N+](=O)[O-] |
Canonical SMILES |
C1=CC(=CC=C1C(=O)[O-])[N+](=O)[O-] |
Origin of Product |
United States |
Synthesis routes and methods
Procedure details
Retrosynthesis Analysis
AI-Powered Synthesis Planning: Our tool employs the Template_relevance Pistachio, Template_relevance Bkms_metabolic, Template_relevance Pistachio_ringbreaker, Template_relevance Reaxys, Template_relevance Reaxys_biocatalysis model, leveraging a vast database of chemical reactions to predict feasible synthetic routes.
One-Step Synthesis Focus: Specifically designed for one-step synthesis, it provides concise and direct routes for your target compounds, streamlining the synthesis process.
Accurate Predictions: Utilizing the extensive PISTACHIO, BKMS_METABOLIC, PISTACHIO_RINGBREAKER, REAXYS, REAXYS_BIOCATALYSIS database, our tool offers high-accuracy predictions, reflecting the latest in chemical research and data.
Strategy Settings
Precursor scoring | Relevance Heuristic |
---|---|
Min. plausibility | 0.01 |
Model | Template_relevance |
Template Set | Pistachio/Bkms_metabolic/Pistachio_ringbreaker/Reaxys/Reaxys_biocatalysis |
Top-N result to add to graph | 6 |
Feasible Synthetic Routes
Disclaimer and Information on In-Vitro Research Products
Please be aware that all articles and product information presented on BenchChem are intended solely for informational purposes. The products available for purchase on BenchChem are specifically designed for in-vitro studies, which are conducted outside of living organisms. In-vitro studies, derived from the Latin term "in glass," involve experiments performed in controlled laboratory settings using cells or tissues. It is important to note that these products are not categorized as medicines or drugs, and they have not received approval from the FDA for the prevention, treatment, or cure of any medical condition, ailment, or disease. We must emphasize that any form of bodily introduction of these products into humans or animals is strictly prohibited by law. It is essential to adhere to these guidelines to ensure compliance with legal and ethical standards in research and experimentation.