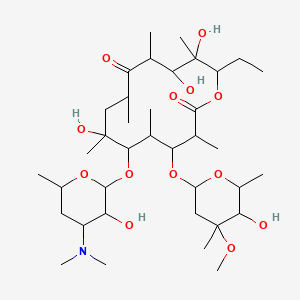
Eritrocina
- Click on QUICK INQUIRY to receive a quote from our team of experts.
- With the quality product at a COMPETITIVE price, you can focus more on your research.
Overview
Description
Erythromycin is a macrolide antibiotic derived from the bacterium Saccharopolyspora erythraea (formerly Streptomyces erythraeus) . It is widely used to treat a variety of bacterial infections, including respiratory tract infections, skin infections, chlamydia infections, pelvic inflammatory disease, and syphilis . Erythromycin works by inhibiting bacterial protein synthesis, making it either bacteriostatic or bactericidal depending on its concentration and the type of microorganism .
Preparation Methods
Erythromycin is primarily produced through microbial fermentation. The bacterium Saccharopolyspora erythraea is cultured in a nutrient-rich medium, where it synthesizes erythromycin as a secondary metabolite . The fermentation broth is then subjected to a series of extraction and purification steps, including solvent extraction, chromatography, and crystallization . Industrial production often involves the use of butyl acetate for extraction and antisolvent crystallization for purification .
Chemical Reactions Analysis
Erythromycin undergoes various chemical reactions, including:
Oxidation: Erythromycin can be oxidized to form erythromycin oxime.
Reduction: Reduction of erythromycin can yield erythromycin amine.
Substitution: Erythromycin can undergo nucleophilic substitution reactions, particularly at the hydroxyl groups.
Common reagents used in these reactions include oxidizing agents like hydrogen peroxide, reducing agents like sodium borohydride, and nucleophiles like amines . The major products formed from these reactions are erythromycin derivatives, which can have different pharmacological properties .
Scientific Research Applications
Erythromycin has a broad range of applications in scientific research:
Mechanism of Action
Erythromycin exerts its effects by binding to the 50S subunit of the bacterial ribosome, thereby inhibiting protein synthesis . This binding blocks the exit tunnel of the ribosome, preventing the elongation of the nascent protein chain . The primary molecular targets are the bacterial ribosomal RNA and associated proteins .
Comparison with Similar Compounds
Erythromycin belongs to the macrolide class of antibiotics, which also includes azithromycin and clarithromycin . Compared to erythromycin, azithromycin and clarithromycin have improved pharmacokinetic profiles, including better acid stability and longer half-lives . These properties make them more effective in certain clinical situations . erythromycin remains unique due to its specific binding affinity and spectrum of activity .
Similar Compounds
- Azithromycin
- Clarithromycin
- Spiramycin
- Roxithromycin
Properties
IUPAC Name |
6-[4-(dimethylamino)-3-hydroxy-6-methyloxan-2-yl]oxy-14-ethyl-7,12,13-trihydroxy-4-(5-hydroxy-4-methoxy-4,6-dimethyloxan-2-yl)oxy-3,5,7,9,11,13-hexamethyl-oxacyclotetradecane-2,10-dione |
Source
|
---|---|---|
Details | Computed by Lexichem TK 2.7.0 (PubChem release 2021.05.07) | |
Source | PubChem | |
URL | https://pubchem.ncbi.nlm.nih.gov | |
Description | Data deposited in or computed by PubChem | |
InChI |
InChI=1S/C37H67NO13/c1-14-25-37(10,45)30(41)20(4)27(39)18(2)16-35(8,44)32(51-34-28(40)24(38(11)12)15-19(3)47-34)21(5)29(22(6)33(43)49-25)50-26-17-36(9,46-13)31(42)23(7)48-26/h18-26,28-32,34,40-42,44-45H,14-17H2,1-13H3 |
Source
|
Details | Computed by InChI 1.0.6 (PubChem release 2021.05.07) | |
Source | PubChem | |
URL | https://pubchem.ncbi.nlm.nih.gov | |
Description | Data deposited in or computed by PubChem | |
InChI Key |
ULGZDMOVFRHVEP-UHFFFAOYSA-N |
Source
|
Details | Computed by InChI 1.0.6 (PubChem release 2021.05.07) | |
Source | PubChem | |
URL | https://pubchem.ncbi.nlm.nih.gov | |
Description | Data deposited in or computed by PubChem | |
Canonical SMILES |
CCC1C(C(C(C(=O)C(CC(C(C(C(C(C(=O)O1)C)OC2CC(C(C(O2)C)O)(C)OC)C)OC3C(C(CC(O3)C)N(C)C)O)(C)O)C)C)O)(C)O |
Source
|
Details | Computed by OEChem 2.3.0 (PubChem release 2021.05.07) | |
Source | PubChem | |
URL | https://pubchem.ncbi.nlm.nih.gov | |
Description | Data deposited in or computed by PubChem | |
Molecular Formula |
C37H67NO13 |
Source
|
Details | Computed by PubChem 2.1 (PubChem release 2021.05.07) | |
Source | PubChem | |
URL | https://pubchem.ncbi.nlm.nih.gov | |
Description | Data deposited in or computed by PubChem | |
DSSTOX Substance ID |
DTXSID90859206 |
Source
|
Record name | 6-{[4-(Dimethylamino)-3-hydroxy-6-methyloxan-2-yl]oxy}-14-ethyl-7,12,13-trihydroxy-4-[(5-hydroxy-4-methoxy-4,6-dimethyloxan-2-yl)oxy]-3,5,7,9,11,13-hexamethyl-1-oxacyclotetradecane-2,10-dione (non-preferred name) | |
Source | EPA DSSTox | |
URL | https://comptox.epa.gov/dashboard/DTXSID90859206 | |
Description | DSSTox provides a high quality public chemistry resource for supporting improved predictive toxicology. | |
Molecular Weight |
733.9 g/mol |
Source
|
Details | Computed by PubChem 2.1 (PubChem release 2021.05.07) | |
Source | PubChem | |
URL | https://pubchem.ncbi.nlm.nih.gov | |
Description | Data deposited in or computed by PubChem | |
CAS No. |
114-07-8 |
Source
|
Record name | erythromycin | |
Source | DTP/NCI | |
URL | https://dtp.cancer.gov/dtpstandard/servlet/dwindex?searchtype=NSC&outputformat=html&searchlist=55929 | |
Description | The NCI Development Therapeutics Program (DTP) provides services and resources to the academic and private-sector research communities worldwide to facilitate the discovery and development of new cancer therapeutic agents. | |
Explanation | Unless otherwise indicated, all text within NCI products is free of copyright and may be reused without our permission. Credit the National Cancer Institute as the source. | |
Disclaimer and Information on In-Vitro Research Products
Please be aware that all articles and product information presented on BenchChem are intended solely for informational purposes. The products available for purchase on BenchChem are specifically designed for in-vitro studies, which are conducted outside of living organisms. In-vitro studies, derived from the Latin term "in glass," involve experiments performed in controlled laboratory settings using cells or tissues. It is important to note that these products are not categorized as medicines or drugs, and they have not received approval from the FDA for the prevention, treatment, or cure of any medical condition, ailment, or disease. We must emphasize that any form of bodily introduction of these products into humans or animals is strictly prohibited by law. It is essential to adhere to these guidelines to ensure compliance with legal and ethical standards in research and experimentation.