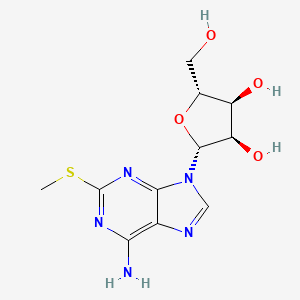
2-Methylthioadenosine
Overview
Description
2-Methylthioadenosine is a naturally occurring sulfur-containing nucleoside found in various species, including prokaryotes, yeast, plants, and higher eukaryotes . It plays a significant role in cellular metabolism and has been studied for its potential therapeutic applications, particularly in cancer and neurological diseases .
Scientific Research Applications
2-Methylthioadenosine has a wide range of applications in scientific research:
Chemistry: It is used as a building block in the synthesis of more complex molecules.
Biology: It plays a role in cellular signaling and metabolism.
Industry: It is used in the production of pharmaceuticals and as a research tool in biochemical studies.
Mechanism of Action
Target of Action
2-Methylthioadenosine (MTA), also known as NSC 36900, primarily targets oligodendrocytes and myofibroblasts . Oligodendrocytes are a type of neuroglial cell in the central nervous system that play a crucial role in the formation of the myelin sheath, which is essential for the proper functioning of the nervous system . Myofibroblasts are cells that play a key role in wound healing and fibrosis .
Mode of Action
MTA interacts with its targets by modulating methyltransferase activity , thereby influencing DNA and protein methylation . In models of neuroinflammation or chemical demyelination, MTA prevents the loss of myelin and promotes remyelination by increasing the number of mature myelinating oligodendrocytes . MTA also inhibits the activation and proliferation of isolated myofibroblasts and down-regulates cyclin D1 gene expression at the transcriptional level .
Biochemical Pathways
MTA is a metabolite of the polyamine pathway . It enhances STAT-3 phosphorylation in astrocytes in vitro, leading to the production of ciliary neurotrophic factor (CNTF), a trophic factor known to promote oligodendrocyte maturation and differentiation, as well as remyelination . MTA’s neuroprotective capacity is also related to its ability to reduce ROS production and oxidative stress .
Pharmacokinetics
The pharmacokinetics of MTA are currently being studied in a first-in-human (FIH), multicenter, open-label, phase 1/1b/2 trial . The primary objectives of this trial include evaluating the safety, tolerability, pharmacokinetics (PK), pharmacodynamics, and efficacy of AMG 193 (a drug related to MTA) in patients with advanced MTAP-null solid tumors .
Result of Action
MTA has been shown to produce neuroprotection in models of inflammation, ischemia, and epilepsy . It prevents the loss of myelin and promotes remyelination, thereby protecting axons in demyelinating diseases and aiding functional recovery . MTA also has anti-oxidant effects and can activate pathways related to protection against stress and production of neurite differentiation .
Action Environment
The action of MTA can be influenced by environmental factors such as the presence of inflammation or chemical demyelination . In these conditions, MTA has been shown to prevent the loss of myelin and promote remyelination . The efficacy and stability of MTA can also be influenced by the presence of oxygen, suggesting that the ecological niche may play an important role for the existence and metabolic steps of the pathway .
Safety and Hazards
Future Directions
The loss of the 5′-methylthioadenosine phosphorylase (MTAP) gene is being studied as a potential therapeutic approach for treating glioblastoma . MTAP encodes a metabolic enzyme required for the metabolism of polyamines and purines leading to DNA synthesis . Another study suggests that enzyme-mediated depletion of methylthioadenosine restores T cell function in MTAP-deficient tumors and reverses immunotherapy resistance .
Biochemical Analysis
Biochemical Properties
2-Methylthioadenosine is known to interact with several enzymes and proteins, influencing various biochemical reactions. One of the key enzymes it interacts with is methylthioadenosine phosphorylase, which catalyzes the phosphorolysis of this compound to adenine and 5-methylthioribose-1-phosphate . This interaction is essential for the methionine salvage pathway, allowing the recycling of methionine from this compound. Additionally, this compound has been shown to inhibit the activity of certain protein arginine methyltransferases, affecting the methylation of arginine residues in proteins .
Cellular Effects
This compound has been observed to exert various effects on different cell types and cellular processes. In melanoma cells, it has been demonstrated to inhibit cell proliferation and induce apoptosis . This compound also influences cell signaling pathways, such as the Akt and S6 ribosomal protein pathways, leading to the down-regulation of cyclin D1 and inhibition of cell cycle progression . Furthermore, this compound affects gene expression by modulating the activity of transcription factors and other regulatory proteins.
Molecular Mechanism
The molecular mechanism of this compound involves its interaction with specific biomolecules and enzymes. It binds to methylthioadenosine phosphorylase, facilitating the breakdown of this compound into adenine and 5-methylthioribose-1-phosphate . This reaction is crucial for the methionine salvage pathway. Additionally, this compound inhibits the activity of protein arginine methyltransferases, leading to reduced methylation of arginine residues in proteins . This inhibition affects various cellular processes, including gene expression and signal transduction.
Temporal Effects in Laboratory Settings
In laboratory settings, the effects of this compound have been studied over time to understand its stability, degradation, and long-term impact on cellular function. Studies have shown that this compound is relatively stable under physiological conditions, but it can undergo degradation in the presence of certain enzymes . Long-term exposure to this compound has been associated with sustained inhibition of cell proliferation and induction of apoptosis in various cell types . These effects are particularly pronounced in cancer cells, where this compound has shown potential as a therapeutic agent.
Dosage Effects in Animal Models
The effects of this compound vary with different dosages in animal models. At lower doses, it has been observed to promote neuroprotection and remyelination in models of inflammation and demyelination . At higher doses, this compound can exhibit toxic effects, including hepatotoxicity and nephrotoxicity . These adverse effects highlight the importance of determining the optimal dosage for therapeutic applications.
Metabolic Pathways
This compound is involved in the methionine salvage pathway, where it is converted to adenine and 5-methylthioribose-1-phosphate by methylthioadenosine phosphorylase . This pathway is essential for the recycling of methionine and the maintenance of cellular methionine levels. Additionally, this compound can influence other metabolic pathways, such as polyamine biosynthesis and the folate cycle, by modulating the availability of key metabolites .
Transport and Distribution
The transport and distribution of this compound within cells and tissues are mediated by specific transporters and binding proteins. It is transported across the plasma membrane by nucleoside transporters, which facilitate its uptake into cells . Once inside the cell, this compound can interact with various binding proteins that influence its localization and accumulation. These interactions are crucial for the proper functioning of this compound in cellular processes.
Subcellular Localization
This compound is localized in various subcellular compartments, including the cytoplasm and nucleus . Its subcellular localization is influenced by targeting signals and post-translational modifications that direct it to specific compartments. In the nucleus, this compound can interact with transcription factors and other regulatory proteins, affecting gene expression and other nuclear processes . In the cytoplasm, it participates in metabolic reactions and signaling pathways that are essential for cellular function.
Preparation Methods
Synthetic Routes and Reaction Conditions: The synthesis of 2-Methylthioadenosine typically involves the methylation of adenosine. One common method includes the reaction of adenosine with methyl iodide in the presence of a base such as sodium hydride . The reaction is carried out in an aprotic solvent like dimethylformamide at elevated temperatures to facilitate the methylation process.
Industrial Production Methods: Industrial production of this compound may involve biotechnological approaches, such as the use of genetically engineered microorganisms to produce the compound. These methods can be more cost-effective and environmentally friendly compared to traditional chemical synthesis .
Chemical Reactions Analysis
Types of Reactions: 2-Methylthioadenosine undergoes various chemical reactions, including:
Oxidation: It can be oxidized to form sulfoxides and sulfones.
Reduction: Reduction reactions can convert it back to its parent nucleoside, adenosine.
Substitution: The methylthio group can be substituted with other functional groups under appropriate conditions.
Common Reagents and Conditions:
Oxidation: Reagents like hydrogen peroxide or m-chloroperbenzoic acid are commonly used.
Reduction: Reducing agents such as lithium aluminum hydride or sodium borohydride are employed.
Substitution: Nucleophilic substitution reactions often use reagents like sodium azide or thiols.
Major Products Formed:
Oxidation: Sulfoxides and sulfones.
Reduction: Adenosine.
Substitution: Various substituted adenosine derivatives.
Comparison with Similar Compounds
Adenosine: The parent nucleoside without the methylthio group.
S-Adenosylmethionine: A related compound involved in methylation reactions.
Methylthioadenosine Phosphorylase: An enzyme that metabolizes 2-Methylthioadenosine.
Uniqueness: this compound is unique due to its sulfur-containing methylthio group, which imparts distinct chemical and biological properties. It has specific roles in cellular metabolism and therapeutic potential that are not shared by its analogs .
Properties
IUPAC Name |
2-(6-amino-2-methylsulfanylpurin-9-yl)-5-(hydroxymethyl)oxolane-3,4-diol | |
---|---|---|
Details | Computed by Lexichem TK 2.7.0 (PubChem release 2021.05.07) | |
Source | PubChem | |
URL | https://pubchem.ncbi.nlm.nih.gov | |
Description | Data deposited in or computed by PubChem | |
InChI |
InChI=1S/C11H15N5O4S/c1-21-11-14-8(12)5-9(15-11)16(3-13-5)10-7(19)6(18)4(2-17)20-10/h3-4,6-7,10,17-19H,2H2,1H3,(H2,12,14,15) | |
Details | Computed by InChI 1.0.6 (PubChem release 2021.05.07) | |
Source | PubChem | |
URL | https://pubchem.ncbi.nlm.nih.gov | |
Description | Data deposited in or computed by PubChem | |
InChI Key |
AJNDEAZTAFKOOO-UHFFFAOYSA-N | |
Details | Computed by InChI 1.0.6 (PubChem release 2021.05.07) | |
Source | PubChem | |
URL | https://pubchem.ncbi.nlm.nih.gov | |
Description | Data deposited in or computed by PubChem | |
Canonical SMILES |
CSC1=NC(=C2C(=N1)N(C=N2)C3C(C(C(O3)CO)O)O)N | |
Details | Computed by OEChem 2.3.0 (PubChem release 2021.05.07) | |
Source | PubChem | |
URL | https://pubchem.ncbi.nlm.nih.gov | |
Description | Data deposited in or computed by PubChem | |
Molecular Formula |
C11H15N5O4S | |
Details | Computed by PubChem 2.1 (PubChem release 2021.05.07) | |
Source | PubChem | |
URL | https://pubchem.ncbi.nlm.nih.gov | |
Description | Data deposited in or computed by PubChem | |
DSSTOX Substance ID |
DTXSID50961410 | |
Record name | 2-(Methylsulfanyl)-9-pentofuranosyl-9H-purin-6-amine | |
Source | EPA DSSTox | |
URL | https://comptox.epa.gov/dashboard/DTXSID50961410 | |
Description | DSSTox provides a high quality public chemistry resource for supporting improved predictive toxicology. | |
Molecular Weight |
313.34 g/mol | |
Details | Computed by PubChem 2.1 (PubChem release 2021.05.07) | |
Source | PubChem | |
URL | https://pubchem.ncbi.nlm.nih.gov | |
Description | Data deposited in or computed by PubChem | |
CAS No. |
4105-39-9 | |
Record name | NSC36900 | |
Source | DTP/NCI | |
URL | https://dtp.cancer.gov/dtpstandard/servlet/dwindex?searchtype=NSC&outputformat=html&searchlist=36900 | |
Description | The NCI Development Therapeutics Program (DTP) provides services and resources to the academic and private-sector research communities worldwide to facilitate the discovery and development of new cancer therapeutic agents. | |
Explanation | Unless otherwise indicated, all text within NCI products is free of copyright and may be reused without our permission. Credit the National Cancer Institute as the source. | |
Record name | 2-(Methylsulfanyl)-9-pentofuranosyl-9H-purin-6-amine | |
Source | EPA DSSTox | |
URL | https://comptox.epa.gov/dashboard/DTXSID50961410 | |
Description | DSSTox provides a high quality public chemistry resource for supporting improved predictive toxicology. | |
Retrosynthesis Analysis
AI-Powered Synthesis Planning: Our tool employs the Template_relevance Pistachio, Template_relevance Bkms_metabolic, Template_relevance Pistachio_ringbreaker, Template_relevance Reaxys, Template_relevance Reaxys_biocatalysis model, leveraging a vast database of chemical reactions to predict feasible synthetic routes.
One-Step Synthesis Focus: Specifically designed for one-step synthesis, it provides concise and direct routes for your target compounds, streamlining the synthesis process.
Accurate Predictions: Utilizing the extensive PISTACHIO, BKMS_METABOLIC, PISTACHIO_RINGBREAKER, REAXYS, REAXYS_BIOCATALYSIS database, our tool offers high-accuracy predictions, reflecting the latest in chemical research and data.
Strategy Settings
Precursor scoring | Relevance Heuristic |
---|---|
Min. plausibility | 0.01 |
Model | Template_relevance |
Template Set | Pistachio/Bkms_metabolic/Pistachio_ringbreaker/Reaxys/Reaxys_biocatalysis |
Top-N result to add to graph | 6 |
Feasible Synthetic Routes
A: 2-methylthioadenosine primarily acts as an agonist or antagonist at purinergic receptors, specifically various subtypes of the P2Y family. [, , , , , , , , , ] Its binding to these receptors triggers a cascade of intracellular signaling events, often involving G proteins and downstream effectors like phospholipase C. [, , , , ] Depending on the specific receptor subtype and cell type, this can lead to diverse physiological responses such as smooth muscle contraction or relaxation, neurotransmitter release, ion channel modulation, and regulation of cellular functions like glycogen metabolism. [, , , , ]
ANone: this compound is a purine nucleoside featuring a methylthio group at the 2-position of the adenine base.
A: this compound is not inherently catalytic. It acts as a ligand for purinergic receptors, modulating their activity rather than directly catalyzing chemical reactions. [, , , , , , , , , ]
A: Yes, computational chemistry techniques have been employed to study this compound, particularly in the context of its interaction with the P2Y1 receptor. [] Molecular docking and molecular dynamics simulations can provide insights into the binding mode of this compound within the receptor's binding pocket, aiding in understanding its structure-activity relationships and potentially guiding the design of novel P2Y1 receptor modulators.
A: Research indicates that modifications to the this compound structure can significantly impact its activity, potency, and selectivity for different P2Y receptor subtypes. [, , ] For instance, the presence of a methylthio group at the 2-position is crucial for its activity at certain P2Y receptors, like the canine P2Y11 receptor. [] Modifications to the phosphate chain, such as replacing oxygen atoms with methylene groups, can also affect potency and the kinetics of receptor interaction. [] Additionally, subtle structural variations can lead to shifts in agonist versus antagonist behavior at the same receptor subtype. []
Disclaimer and Information on In-Vitro Research Products
Please be aware that all articles and product information presented on BenchChem are intended solely for informational purposes. The products available for purchase on BenchChem are specifically designed for in-vitro studies, which are conducted outside of living organisms. In-vitro studies, derived from the Latin term "in glass," involve experiments performed in controlled laboratory settings using cells or tissues. It is important to note that these products are not categorized as medicines or drugs, and they have not received approval from the FDA for the prevention, treatment, or cure of any medical condition, ailment, or disease. We must emphasize that any form of bodily introduction of these products into humans or animals is strictly prohibited by law. It is essential to adhere to these guidelines to ensure compliance with legal and ethical standards in research and experimentation.