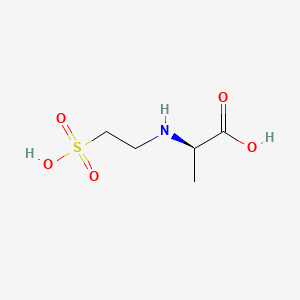
Tauropine
Overview
Description
It is a type of opine, which are anaerobic metabolites found in marine invertebrates such as abalone and certain algae . Tauropine is produced through the reductive condensation of taurine and pyruvate, catalyzed by the enzyme this compound dehydrogenase . This compound plays a significant role in the anaerobic metabolism of marine organisms.
Preparation Methods
Synthetic Routes and Reaction Conditions: Tauropine can be synthesized by reacting taurine with L-bromopropionic acid in a 1M NaOH solution for seven days . The reaction mixture is then applied to a Dowex 1-X2 column, washed with water and 0.1M HCl, and eluted with 0.2M HCl. The eluant is concentrated using a rotary evaporator, and the residue is recrystallized with ethanol and lyophilized .
Industrial Production Methods: The use of high-performance liquid chromatography (HPLC) and mass spectrometry (MS) can ensure the purity and yield of the compound .
Chemical Reactions Analysis
Tauropine Dehydrogenase Reaction
-
Reaction Type : this compound dehydrogenase (EC 1.5.1.23) is an enzyme that catalyzes a reversible oxidoreduction reaction .
-
Reactants : The substrates for this reaction are taurine, pyruvate, NADH, and H+ .
-
Products : The products of this enzyme are this compound, NAD+, and H2O .
-
Enzyme Class : this compound dehydrogenase belongs to the family of oxidoreductases, specifically those acting on the CH-NH group of donors with NAD+ or NADP+ as acceptor .
-
Systematic Name : N2-(D-1-carboxyethyl) taurine: NAD+ oxidoreductase (taurine-forming) is the systematic name of this enzyme class .
Role of Taurine in Chemical Reactions
Taurine is involved in various chemical reactions due to its reactivity with aldehydes and its role as an antioxidant .
-
Reactivity with Aldehydes : Taurine reacts with aldehydes such as glucose, acetaldehyde, and malondialdehyde. It demonstrates high reactivity with each of these aldehydes .
-
Inhibiting Protein Modification : Taurine can inhibit the modification of proteins by aldehydes. For example, it shows an inhibiting effect against the modification of low-density lipoprotein (LDL) by malondialdehyde .
-
Antioxidative Effect : Taurine-glucose reaction products exhibit an antioxidative effect on the peroxidation of liposomes, suggesting a potential antioxidative role in vivo through reactions with aldehydes .
-
Antioxidant Mechanisms : Taurine acts as a natural antioxidant through various mechanisms :
-
Scavenging free radicals like peroxyl radicals, superoxide anions, nitric oxide, and peroxynitrite.
-
Binding metal ions.
-
Decomposing peroxides.
-
Breaking chains by scavenging intermediate radicals.
-
Binding reactive products of peroxidation.
-
Derivatization of Bile Acids with Taurine
Taurine is used in the derivatization of bile acids for analysis by fast atom bombardment mass spectrometry .
-
Method : A method has been developed for rapid coupling of taurine to unconjugated, glycine-conjugated, sulfated, and glucuronidated bile acids .
-
Reaction Conditions : The reaction is performed for 2 hours at room temperature in an aqueous pyridine hydrochloride buffer, with or without dioxane, using 0.1 M 1-ethyl-3-(3-dimethylaminopropyl) carbodiimide as the coupling agent and 0.2 M taurine .
-
Yields : The yields of this reaction are typically higher than 95% .
-
Advantage : This method allows conjugation of bile acids with the labile 7 alpha-hydroxy-3-oxo-4-ene structure .
Chlorination of Taurine by Myeloperoxidase
Taurine undergoes chlorination by the myeloperoxidase-H2O2-Cl- system .
-
Mechanism : The chlorination reaction involves an enzyme-bound chlorinating intermediate .
-
Kinetic Parameters : The kinetic parameters at pH 4.7 are k1 = (3.3 +/- 0.2) x 107 M-1 S-1, k2 = (2.8 +/- 1.2) x 106 M-1 S-1, and k3 = (4.4 +/- 0.2) x 105 M-1 S-1 .
-
Role in Neutrophils : Taurine plays a role in protecting certain targets, including myeloperoxidase, from potent chlorinated oxidants by forming taurine monochloramine, a relatively non-toxic and stable compound .
N-Acetyltaurine Breakdown
N-acetyltaurine can be broken down into its component parts, acetate, and taurine, by the enzyme PTER .
Scientific Research Applications
Biochemical Applications
1.1 Enzymatic Role
Tauropine dehydrogenase is an enzyme that catalyzes the conversion of this compound to taurine and vice versa. This enzyme has been isolated from various marine organisms, such as the sponge Halichondria japonica, where it plays a crucial role in the organism's metabolic processes under anaerobic conditions . Understanding the kinetics and mechanisms of this compound dehydrogenase can provide insights into metabolic pathways in marine ecosystems.
1.2 Metabolic Indicator
Research indicates that this compound accumulates as an end product of anaerobic metabolism in certain marine species, such as mollusks. Its levels can serve as an early indicator of metabolic stress during anoxia, making it a valuable biomarker for ecological studies . This application is particularly relevant in assessing the health of marine environments affected by hypoxic conditions.
Pharmacological Insights
2.1 Bioavailability Studies
A study investigating the pharmacokinetics of this compound revealed that its bioavailability after oral ingestion is limited, as it was not detectable in the blood of orally treated mice . This finding suggests that while this compound may have potential therapeutic properties, its delivery methods need optimization for effective absorption.
2.2 Potential Therapeutic Uses
Given its structural similarity to taurine, this compound may exhibit similar neuroprotective and antioxidant properties. Future research could explore its efficacy in treating neurological disorders or oxidative stress-related conditions.
Environmental Applications
3.1 Impact on Marine Life
The expression of this compound dehydrogenase has been linked to stress responses in marine organisms exposed to environmental changes such as ocean acidification and pollutants like oxybenzone . Understanding how this compound functions under these stressors can help develop strategies for conserving marine biodiversity.
3.2 Biomarker for Pollution Monitoring
Due to its role in metabolic processes affected by environmental stressors, this compound has potential as a biomarker for monitoring pollution levels in marine ecosystems. Its accumulation can indicate the physiological state of organisms exposed to contaminants.
Table 1: Summary of Research Findings on this compound Applications
Mechanism of Action
Tauropine exerts its effects primarily through its role in anaerobic metabolism. The enzyme this compound dehydrogenase catalyzes the conversion of taurine and pyruvate to this compound, which helps maintain redox balance under hypoxic conditions . This process is crucial for the survival of marine organisms in low-oxygen environments .
Comparison with Similar Compounds
Octopine: Another opine found in marine invertebrates, produced from arginine and pyruvate.
Strombine: Formed from glycine and pyruvate, also found in marine organisms.
Alanopine: Produced from alanine and pyruvate.
Uniqueness of Tauropine: this compound is unique due to its specific formation from taurine and pyruvate, catalyzed by this compound dehydrogenase . Its presence in marine invertebrates and algae, along with its potential health benefits, sets it apart from other opines .
Biological Activity
Tauropine, a non-proteinogenic amino acid derivative, is primarily known for its role in the anaerobic metabolism of certain marine organisms, particularly in the context of hypoxia. It is synthesized through the action of this compound dehydrogenase (TaDH), which catalyzes the reduction of pyruvate using taurine as a substrate alongside NADH. This article explores the biological activity of this compound, focusing on its enzymatic properties, physiological roles, and implications in metabolic processes.
Enzymatic Properties of this compound Dehydrogenase
This compound dehydrogenase is a key enzyme that facilitates the conversion of pyruvate and taurine into this compound. The enzyme has been purified from the shell adductor muscle of the ormer (Haliotis lamellosa), revealing several important characteristics:
- Molecular Weight : The enzyme exhibits a relative molecular mass of approximately 38,000 Da when assessed by gel filtration and 42,000 Da via SDS-PAGE.
- Substrate Specificity : The preferred substrates for TaDH are this compound and pyruvate, with alanine being a less effective alternative substrate.
- Kinetic Parameters : The apparent Km values for NADH, pyruvate, and taurine are 0.022 mM, 0.64 mM, and 64.7 mM respectively at pH 7.0. Product inhibition studies indicate that both NAD+ and this compound inhibit the forward reaction, with NAD+ acting as a competitive inhibitor .
Physiological Role
The physiological significance of this compound and its dehydrogenase has been extensively studied in various organisms under anaerobic conditions. In marine species like Haliotis lamellosa, this compound serves as an important metabolic intermediate during periods of low oxygen availability.
- Metabolic Pathway : During anaerobiosis, organisms utilize this compound to regenerate NAD+, which is crucial for maintaining glycolytic flux and energy production.
- Hypoxia Response : Research indicates that genes related to this compound metabolism are upregulated in response to hypoxic conditions, suggesting a protective role against oxidative stress .
Case Studies and Research Findings
Several studies have documented the effects of this compound in different biological contexts:
- Marine Organisms : A study on deep-sea corals revealed that this compound dehydrogenase expression is significantly increased under hypoxic conditions, indicating its role in redox balance and energy metabolism during stress .
- Toxicology Studies : Investigations into the interactions between environmental toxins and this compound metabolism have shown that exposure to certain pollutants can alter the expression of genes involved in this compound synthesis, affecting overall metabolic health .
- Comparative Studies : Research comparing different marine species has highlighted variations in this compound levels based on environmental oxygen availability, demonstrating its adaptive significance in diverse habitats .
Data Table: Kinetic Parameters of this compound Dehydrogenase
Substrate | Apparent Km (mM) | Specific Activity (units/mg) |
---|---|---|
NADH | 0.022 ± 0.003 | 463 |
Pyruvate | 0.64 ± 0.07 | - |
Taurine | 64.7 ± 5.4 | - |
Q & A
Basic Research Questions
Q. How is tauropine detected and quantified in biological samples, and what methodological considerations ensure accuracy?
this compound detection typically employs high-performance liquid chromatography (HPLC) paired with mass spectrometry (MS) or nuclear magnetic resonance (NMR) spectroscopy. Key considerations include:
- Sample preparation : Avoid degradation by flash-freezing tissues (e.g., abalone foot muscle) and using protease inhibitors .
- Calibration standards : Use synthetic this compound to validate retention times and spectral profiles.
- Reproducibility : Include internal controls (e.g., deuterated analogs) to account for matrix effects . Example: In abalone studies, this compound levels correlated with lactate under anaerobic stress, requiring simultaneous measurement of both metabolites to avoid cross-reactivity .
Q. What is the biological role of this compound in marine invertebrates under environmental stress?
this compound functions as an anaerobic end-product, accumulating during oxygen-limited conditions (e.g., aerial exposure in abalone). Methodological insights:
- Experimental stress induction : Simulate transport or emersion stress at controlled temperatures (e.g., 12–24 h at 15°C) to replicate field conditions .
- Temporal resolution : Measure this compound at intervals (e.g., 0 h, 12 h, 24 h) to track metabolic shifts.
- Comparative analysis : Contrast this compound levels with other osmolytes (e.g., betaine) to disentangle stress-specific responses .
Advanced Research Questions
Q. How can experimental designs address contradictions in this compound accumulation data across species or conditions?
Contradictions often arise from interspecies variability (e.g., Haliotis vs. Perna species) or methodological disparities. Strategies include:
- Standardized protocols : Adopt FINER criteria (Feasible, Interesting, Novel, Ethical, Relevant) to harmonize stress induction and sampling .
- Meta-analysis : Aggregate data from studies using identical detection methods (e.g., HPLC-MS) and normalize to tissue mass/volume . Example: Pre-cooled abalone showed earlier this compound peaks (12 h) versus non-cooled groups (24 h), highlighting thermal acclimation effects .
Q. What metabolic pathways interact with this compound production, and how can pathway inhibition/activation studies clarify its regulation?
this compound synthesis links to glycolysis and alanine metabolism. Methodological approaches:
- Enzyme assays : Quantify this compound synthase activity under varying pH/temperature to identify kinetic bottlenecks.
- Isotopic labeling : Use -glucose to trace carbon flux into this compound during anaerobic respiration .
- Gene silencing : Knock down this compound-related genes (e.g., tauS) in model organisms to assess compensatory pathways .
Q. How do researchers reconcile conflicting data on this compound’s role in pH homeostasis versus oxidative stress mitigation?
Contradictory findings may stem from:
- Tissue specificity : Compare muscle vs. hepatopancreas this compound levels, as pH buffering capacity varies by organ .
- Omics integration : Pair metabolomic data with transcriptomic profiles of pH-regulatory genes (e.g., V-ATPase) .
- In vitro validation : Test this compound’s antioxidant capacity via radical scavenging assays (e.g., DPPH) at physiological concentrations .
Q. Methodological Rigor and Reproducibility
Q. What steps ensure reproducibility in this compound studies, particularly when replicating field conditions in lab settings?
- Environmental controls : Monitor dissolved oxygen, salinity, and temperature during stress simulations using data loggers .
- Blinded analysis : Assign sample IDs randomly to prevent bias in HPLC/NMR data interpretation .
- Open data : Share raw chromatograms and NMR spectra in supplementary materials to enable cross-validation .
Q. How can researchers design longitudinal studies to assess this compound’s chronic effects on organismal fitness?
- Cohort tracking : Tag individuals (e.g., abalone) and measure this compound post-recovery to evaluate metabolic resilience .
- Fitness proxies : Correlate this compound levels with growth rates, survival, or reproductive output over multiple cycles of stress .
Q. Future Directions
Q. What gaps exist in understanding this compound’s ecological and evolutionary significance, and how can interdisciplinary approaches address them?
Properties
IUPAC Name |
(2R)-2-(2-sulfoethylamino)propanoic acid | |
---|---|---|
Source | PubChem | |
URL | https://pubchem.ncbi.nlm.nih.gov | |
Description | Data deposited in or computed by PubChem | |
InChI |
InChI=1S/C5H11NO5S/c1-4(5(7)8)6-2-3-12(9,10)11/h4,6H,2-3H2,1H3,(H,7,8)(H,9,10,11)/t4-/m1/s1 | |
Source | PubChem | |
URL | https://pubchem.ncbi.nlm.nih.gov | |
Description | Data deposited in or computed by PubChem | |
InChI Key |
VHYQDLYSULDZSO-SCSAIBSYSA-N | |
Source | PubChem | |
URL | https://pubchem.ncbi.nlm.nih.gov | |
Description | Data deposited in or computed by PubChem | |
Canonical SMILES |
CC(C(=O)O)NCCS(=O)(=O)O | |
Source | PubChem | |
URL | https://pubchem.ncbi.nlm.nih.gov | |
Description | Data deposited in or computed by PubChem | |
Isomeric SMILES |
C[C@H](C(=O)O)NCCS(=O)(=O)O | |
Source | PubChem | |
URL | https://pubchem.ncbi.nlm.nih.gov | |
Description | Data deposited in or computed by PubChem | |
Molecular Formula |
C5H11NO5S | |
Source | PubChem | |
URL | https://pubchem.ncbi.nlm.nih.gov | |
Description | Data deposited in or computed by PubChem | |
DSSTOX Substance ID |
DTXSID60955123 | |
Record name | N-(2-Sulfoethyl)alanine | |
Source | EPA DSSTox | |
URL | https://comptox.epa.gov/dashboard/DTXSID60955123 | |
Description | DSSTox provides a high quality public chemistry resource for supporting improved predictive toxicology. | |
Molecular Weight |
197.21 g/mol | |
Source | PubChem | |
URL | https://pubchem.ncbi.nlm.nih.gov | |
Description | Data deposited in or computed by PubChem | |
CAS No. |
33497-79-9 | |
Record name | Tauropine | |
Source | CAS Common Chemistry | |
URL | https://commonchemistry.cas.org/detail?cas_rn=33497-79-9 | |
Description | CAS Common Chemistry is an open community resource for accessing chemical information. Nearly 500,000 chemical substances from CAS REGISTRY cover areas of community interest, including common and frequently regulated chemicals, and those relevant to high school and undergraduate chemistry classes. This chemical information, curated by our expert scientists, is provided in alignment with our mission as a division of the American Chemical Society. | |
Explanation | The data from CAS Common Chemistry is provided under a CC-BY-NC 4.0 license, unless otherwise stated. | |
Record name | Tauropine | |
Source | ChemIDplus | |
URL | https://pubchem.ncbi.nlm.nih.gov/substance/?source=chemidplus&sourceid=0033497799 | |
Description | ChemIDplus is a free, web search system that provides access to the structure and nomenclature authority files used for the identification of chemical substances cited in National Library of Medicine (NLM) databases, including the TOXNET system. | |
Record name | N-(2-Sulfoethyl)alanine | |
Source | EPA DSSTox | |
URL | https://comptox.epa.gov/dashboard/DTXSID60955123 | |
Description | DSSTox provides a high quality public chemistry resource for supporting improved predictive toxicology. | |
Retrosynthesis Analysis
AI-Powered Synthesis Planning: Our tool employs the Template_relevance Pistachio, Template_relevance Bkms_metabolic, Template_relevance Pistachio_ringbreaker, Template_relevance Reaxys, Template_relevance Reaxys_biocatalysis model, leveraging a vast database of chemical reactions to predict feasible synthetic routes.
One-Step Synthesis Focus: Specifically designed for one-step synthesis, it provides concise and direct routes for your target compounds, streamlining the synthesis process.
Accurate Predictions: Utilizing the extensive PISTACHIO, BKMS_METABOLIC, PISTACHIO_RINGBREAKER, REAXYS, REAXYS_BIOCATALYSIS database, our tool offers high-accuracy predictions, reflecting the latest in chemical research and data.
Strategy Settings
Precursor scoring | Relevance Heuristic |
---|---|
Min. plausibility | 0.01 |
Model | Template_relevance |
Template Set | Pistachio/Bkms_metabolic/Pistachio_ringbreaker/Reaxys/Reaxys_biocatalysis |
Top-N result to add to graph | 6 |
Feasible Synthetic Routes
Disclaimer and Information on In-Vitro Research Products
Please be aware that all articles and product information presented on BenchChem are intended solely for informational purposes. The products available for purchase on BenchChem are specifically designed for in-vitro studies, which are conducted outside of living organisms. In-vitro studies, derived from the Latin term "in glass," involve experiments performed in controlled laboratory settings using cells or tissues. It is important to note that these products are not categorized as medicines or drugs, and they have not received approval from the FDA for the prevention, treatment, or cure of any medical condition, ailment, or disease. We must emphasize that any form of bodily introduction of these products into humans or animals is strictly prohibited by law. It is essential to adhere to these guidelines to ensure compliance with legal and ethical standards in research and experimentation.