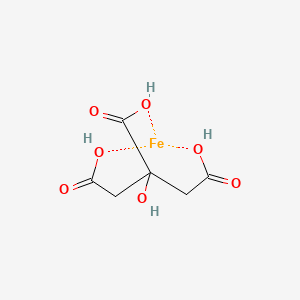
Iron citrate
Overview
Description
Iron citrate, also known as ferric citrate, is a coordination complex formed by the interaction of ferric ions (Fe³⁺) with citric acid. This compound is typically found in the form of a dark orange-red to brown solid and is soluble in water. This compound plays a significant role in various biological and industrial processes due to its ability to solubilize iron, making it bioavailable for various organisms .
Mechanism of Action
Target of Action
Iron citrate, also known as ferric citrate, is primarily targeted towards the erythrocytes, or red blood cells, in the body . Iron is an essential component of hemoglobin, the molecule in red blood cells that carries oxygen from the lungs to the body’s tissues . Therefore, this compound plays a crucial role in oxygen transport and storage .
Mode of Action
This compound acts by forming an insoluble compound with phosphate present in the diet, thus minimizing its uptake by the digestive system . Upon ingestion, ferric iron in this compound is converted to ferrous iron by the enzyme ferric reductase in the gastrointestinal tract . The molecules are absorbed in the same highly regulated physiological pathway as dietary iron . In the bloodstream, ferrous iron is oxidized back to ferric iron, which binds to transferrin, an iron transfer protein that transports iron to the bone marrow for incorporation into hemoglobin for red blood cell production .
Biochemical Pathways
This compound affects several biochemical pathways. It plays a key role in the enzymatic processes of bacteria, plants, and mammals . This compound complexes are considered among the most significant coordinated forms of ferric iron that take place in biochemical processes of all living organisms . This compound is known to facilitate the bioavailability of iron and enhance iron transport through the cell membranes .
Pharmacokinetics
The pharmacokinetics of this compound involves its absorption, distribution, metabolism, and excretion (ADME) properties . Iron can be administered intravenously in the form of polynuclear iron(III)-hydroxide complexes with carbohydrate ligands or orally as iron(II) (ferrous) salts or iron(III) (ferric) complexes . High oral iron doses or rapid release of iron from intravenous iron preparations can saturate the iron transport system, resulting in oxidative stress with adverse clinical and subclinical consequences .
Result of Action
The action of this compound results in the mobilization of cellular iron, reduction of reactive oxygen species (ROS), and restoration of insulin production at clinically achievable levels . Excessive iron can lead to the production and accumulation of dangerous reactive oxygen species and free radicals that can cause considerable damages to most cellular components .
Action Environment
Environmental factors can influence the action of this compound. Iron homeostasis in plants and microbes has to be fine-tuned to maintain fitness . Both plants and microbes have evolved sophisticated mechanisms to modulate iron status at a moderate level for maintaining their fitness . In addition, the physical and chemical speciation of iron, as well as rhizosphere activity, are key factors controlling the bioavailability of iron .
Biochemical Analysis
Biochemical Properties
Iron citrate is known for its ability to form stable complexes with ferric ions, facilitating the solubilization and transport of iron in biological systems. It interacts with several enzymes, proteins, and other biomolecules. For instance, this compound is involved in the iron uptake processes of bacteria such as Escherichia coli, where it forms multiple this compound complexes . In plants, this compound is crucial for the root-to-shoot transport of iron in the xylem . The interaction of this compound with these biomolecules is essential for maintaining iron homeostasis and ensuring the availability of iron for various metabolic processes.
Cellular Effects
This compound influences various cellular processes, including cell signaling pathways, gene expression, and cellular metabolism. In bacterial cells, this compound complexes are utilized for iron uptake, which is vital for their growth and survival . In plants, this compound facilitates the transport of iron from roots to shoots, ensuring proper iron distribution within the plant . This transport is essential for processes such as photosynthesis and respiration. In mammalian cells, this compound plays a role in iron metabolism, impacting cellular functions such as oxygen transport and energy production.
Molecular Mechanism
The molecular mechanism of this compound involves its binding interactions with biomolecules, enzyme activation, and changes in gene expression. This compound forms stable complexes with ferric ions, which are then transported across cell membranes. In bacteria, this compound complexes are recognized by specific transporters that facilitate their uptake . In plants, this compound is transported through the xylem, where it interacts with various proteins to ensure efficient iron delivery . These interactions are crucial for maintaining iron homeostasis and supporting cellular functions.
Temporal Effects in Laboratory Settings
In laboratory settings, the effects of this compound can change over time due to its stability and degradation. Studies have shown that this compound complexes can remain stable in aqueous solutions, but their stability may be affected by factors such as pH and the presence of organic solvents . Long-term effects of this compound on cellular function have been observed in both in vitro and in vivo studies, indicating its importance in maintaining iron homeostasis over extended periods.
Dosage Effects in Animal Models
The effects of this compound vary with different dosages in animal models. At low doses, this compound can enhance iron uptake and improve iron metabolism. At high doses, it may lead to toxic effects due to the accumulation of excess iron . Studies have shown that there are threshold effects, where a certain dosage is required to achieve the desired biological response without causing adverse effects.
Metabolic Pathways
This compound is involved in several metabolic pathways, including those related to iron metabolism and energy production. It interacts with enzymes such as aconitase, which catalyzes the interconversion of citrate and isocitrate . This compound also affects metabolic flux and metabolite levels, influencing processes such as the citric acid cycle and oxidative phosphorylation. These interactions are essential for maintaining cellular energy balance and supporting metabolic functions.
Transport and Distribution
This compound is transported and distributed within cells and tissues through specific transporters and binding proteins. In bacteria, this compound complexes are recognized by transporters that facilitate their uptake . In plants, this compound is transported through the xylem, where it interacts with proteins to ensure efficient iron delivery . In mammalian cells, this compound is distributed to various tissues, where it plays a role in iron metabolism and cellular functions.
Subcellular Localization
The subcellular localization of this compound is influenced by targeting signals and post-translational modifications. In bacterial cells, this compound complexes are localized to specific compartments involved in iron uptake and metabolism . In plant cells, this compound is transported to the xylem and other tissues, where it supports iron distribution and cellular functions . In mammalian cells, this compound is localized to organelles such as mitochondria, where it plays a role in energy production and iron metabolism.
Preparation Methods
Synthetic Routes and Reaction Conditions: Iron citrate can be synthesized through several methods. One common laboratory method involves the reaction of ferric hydroxide with citric acid. The reaction is typically carried out in an aqueous medium, and the mixture is heated to facilitate the formation of this compound. The general reaction is as follows: [ \text{Fe(OH)}_3 + \text{C}_6\text{H}_8\text{O}_7 \rightarrow \text{Fe(C}_6\text{H}_5\text{O}_7) + 3\text{H}_2\text{O} ]
Industrial Production Methods: In industrial settings, this compound is often produced through a one-pot process that involves preparing a ferric hydroxide slurry followed by treatment with a citrate ion source. The reaction mixture is heated to a temperature range of 80-100°C and maintained for 1 to 4 hours to obtain a clear solution of pharmaceutical-grade ferric citrate .
Chemical Reactions Analysis
Types of Reactions: Iron citrate undergoes various chemical reactions, including:
Oxidation-Reduction Reactions: this compound can be photoreduced by exposure to light, especially blue and ultraviolet light, resulting in the reduction of Fe³⁺ to Fe²⁺ and the concomitant oxidation of the carboxyl group adjacent to the hydroxyl group, yielding carbon dioxide and acetonedicarboxylate.
Complexation Reactions: this compound forms various coordination complexes with ferric ions, which can be oligomers or polymers.
Common Reagents and Conditions:
Reagents: Citric acid, ferric hydroxide, and water are commonly used reagents in the synthesis of this compound.
Conditions: The reactions are typically carried out in aqueous solutions, with heating to facilitate the formation of the desired complexes.
Major Products:
Scientific Research Applications
Comparison with Similar Compounds
Iron citrate can be compared with other iron-containing compounds, such as:
Iron(II) Citrate: Unlike this compound, iron(II) citrate contains Fe²⁺ ions and is less stable in air, converting to ferric citrate upon exposure.
Ferric Ammonium Citrate: This compound is similar to this compound but contains ammonium ions.
Ferrous Sulfate: Ferrous sulfate is another common iron supplement, but it contains Fe²⁺ ions and is primarily used to treat iron deficiency anemia.
This compound is unique due to its ability to form various coordination complexes with ferric ions and its role in both biological and industrial processes.
Properties
Key on ui mechanism of action |
... Iron loading by 24-hour incubation with 0.36 mmol/L ferric ammonium citrate resulted in a decrease in the activity of nicotinamide adenine dinucleotide (NADH)-cytochrome c oxidoreductase (complex I+III) to 35.3%+/-11.2% of the value in untreated controls; of succinate-cytochrome c oxidoreductase (complex II+III) to 57.4%+/-3.1%; and of succinate dehydrogenase to 63.5%+/-12.6% (p < 0.001 in all cases). The decrease in activity of other mitochondrial enzymes, including NADH-ferricyanide reductase, succinate ubiquinone oxidoreductase (complex II), cytochrome c oxidase (complex IV), and ubiquinol cytochrome c oxidoreductase (complex III), was less impressive and ranged from 71.5%+/-15.8% to 91.5%+/-14.6% of controls. That the observed loss of respiratory enzyme activity was a specific effect of iron toxicity was clearly demonstrated by the complete restoration of enzyme activities by in vitro iron chelation therapy. Sequential treatment with iron and doxorubicin caused a loss of complex I+III and complex II+III activity that was greater than that seen with either agent alone but was only partially correctable by DF treatment. Alterations in cellular adenosine triphosphate measurements paralleled very closely the changes observed in respiratory complex activity. |
---|---|
CAS No. |
2338-05-8 |
Molecular Formula |
C12H11Fe2O14 |
Molecular Weight |
490.90 g/mol |
IUPAC Name |
3-carboxy-3-hydroxypentanedioate;2-hydroxypropane-1,2,3-tricarboxylate;iron(2+);iron(3+) |
InChI |
InChI=1S/2C6H8O7.2Fe/c2*7-3(8)1-6(13,5(11)12)2-4(9)10;;/h2*13H,1-2H2,(H,7,8)(H,9,10)(H,11,12);;/q;;+2;+3/p-5 |
InChI Key |
YMMYBYGJYFDNIY-UHFFFAOYSA-I |
SMILES |
C(C(=O)O)C(CC(=O)O)(C(=O)O)O.[Fe] |
Canonical SMILES |
C(C(=O)[O-])C(CC(=O)[O-])(C(=O)O)O.C(C(=O)[O-])C(CC(=O)[O-])(C(=O)[O-])O.[Fe+2].[Fe+3] |
density |
1.8 at 68 °F (USCG, 1999) - Denser than water; will sink Specific gravity: 1.8 at 20 °C/4 °C (solid) |
Key on ui other cas no. |
2338-05-8 |
physical_description |
Ferric ammonium citrate is a yellowish brown to red solid with a faint odor of ammonia. It is soluble in water. The primary hazard is the threat to the environment. Immediate steps should be taken to limit its spread to the environment. It is used in medicine, in making blueprints, and as a feed additive. Exists in two forms; Brown hydrated form is garnet-red or brownish-yellow solid; Green hydrated form is green deliquescent solid; Soluble in water; [Merck Index] Brown crystalline solid; [MSDSonline] |
Pictograms |
Irritant |
Related CAS |
3522-50-7 (Parent) 2338-05-8 (unspecified iron salt) 28633-45-6 (unspecified iron(+3) salt) 57979-58-5 (dihydrate) 17217-76-4 (trihydrate) 20074-52-6 (Parent) 77-92-9 (Parent) |
Synonyms |
ferric citrate ferric citrate anhydrous ferric citrate dihydrate ferric citrate hydrate ferric citrate iron(+3) salt ferric citrate trihydrate ferric citrate, 59Fe-labeled cpd ferric citrate, iron salt, 59Fe-labeled cpd ferric-citric acid iron(III) citrate JTT-751 zerenex |
Origin of Product |
United States |
Retrosynthesis Analysis
AI-Powered Synthesis Planning: Our tool employs the Template_relevance Pistachio, Template_relevance Bkms_metabolic, Template_relevance Pistachio_ringbreaker, Template_relevance Reaxys, Template_relevance Reaxys_biocatalysis model, leveraging a vast database of chemical reactions to predict feasible synthetic routes.
One-Step Synthesis Focus: Specifically designed for one-step synthesis, it provides concise and direct routes for your target compounds, streamlining the synthesis process.
Accurate Predictions: Utilizing the extensive PISTACHIO, BKMS_METABOLIC, PISTACHIO_RINGBREAKER, REAXYS, REAXYS_BIOCATALYSIS database, our tool offers high-accuracy predictions, reflecting the latest in chemical research and data.
Strategy Settings
Precursor scoring | Relevance Heuristic |
---|---|
Min. plausibility | 0.01 |
Model | Template_relevance |
Template Set | Pistachio/Bkms_metabolic/Pistachio_ringbreaker/Reaxys/Reaxys_biocatalysis |
Top-N result to add to graph | 6 |
Feasible Synthetic Routes
Q1: How does iron citrate affect iron uptake in human cells?
A: this compound can inhibit the uptake of transferrin-bound iron, the primary iron transport protein, in human cells. This occurs through competitive inhibition, where this compound interferes with the cellular uptake of transferrin-bound iron, primarily through transferrin receptor-independent pathways. []
Q2: Can this compound contribute to iron overload diseases?
A: Research suggests that in the presence of hydrogen peroxide (H2O2), this compound complexes can participate in redox cycling, potentially contributing to the pathophysiological outcomes of iron overload disorders. This redox cycling leads to the generation of reactive oxygen species, potentially damaging cellular components. []
Q3: What is the role of this compound in the context of pyoverdin siderophores?
A: Pyoverdins, siderophores produced by certain bacteria, have been shown to protect human hepatocytes from iron toxicity induced by this compound. They achieve this by both hindering iron uptake and promoting its release from iron-loaded cells. []
Q4: What is the molecular formula of this compound?
A4: this compound exists in various forms. The most common form is ferric citrate, with a molecular formula of C6H5FeO7.
Q5: Is there a method for preparing pharmaceutical-grade this compound?
A: Pharmaceutical-grade this compound can be obtained from conventional this compound by refining it with a polar organic solvent. This solvent is chosen based on its inability to dissolve this compound. []
Q6: What is the impact of this compound on the growth of different cyanobacteria species?
A: Studies show that this compound can have varying effects on cyanobacteria growth. For example, this compound significantly suppresses the growth of Oscillatoria tennis, even at high iron concentrations. In contrast, Anabaena macrospora and Phormidium tenue exhibit better growth with non-autoclaved this compound. Interestingly, Oscillatoria brevis demonstrates the ability to grow even at low iron levels, utilizing various iron forms including this compound. []
Q7: How does this compound affect the proliferation and differentiation of bone cells?
A: Research suggests that this compound can inhibit the proliferation of rat bone marrow mesenchymal stem cells (BMSCs) in a dose-dependent manner. Furthermore, it suppresses the osteogenic differentiation of these cells, evidenced by decreased calcium nodule formation and reduced expression of osteogenic genes. []
Q8: How does this compound influence vascular calcification?
A: Studies indicate that this compound can impede vascular calcification induced by high phosphate levels in vascular smooth muscle cells. This effect is attributed to this compound's ability to both suppress apoptosis, a programmed cell death mechanism, and promote autophagy, a cellular recycling process. [, ]
Q9: Can this compound be used as a precursor for nanoparticle synthesis?
A: Yes, this compound can act as a precursor for synthesizing carbon-encapsulated iron nanoparticles through pyrolysis. The ratio of this compound to carbon sources significantly impacts the yield, crystallinity, morphology, and magnetic properties of the resulting nanoparticles. []
Q10: Have computational methods been used to study this compound?
A10: Although not extensively covered in the provided papers, computational chemistry techniques like density functional theory (DFT) could be used to explore the electronic structure of this compound complexes. Molecular dynamics simulations could provide insights into the behavior of this compound in different solvents and its interactions with biological molecules.
Q11: How do structural variations in iron chelators impact their biological effects?
A: Research on structurally similar iron chelators, like desferrioxamine and deferiprone, reveals that these compounds can inhibit the proliferation of bone cells. This suggests a potential link between iron chelation and its impact on bone cell growth. []
Q12: How does the form of iron influence its effect on ferritin synthesis?
A: Studies on human mononuclear cells suggest that different forms of iron, including this compound, have varying effects on ferritin synthesis. This variation likely stems from differences in their susceptibility to polymerization in aqueous solutions, highlighting the importance of formulation in iron delivery. []
Q13: Can Caco-2 cells be used to study iron bioavailability?
A: Caco-2 cells, derived from a human colon adenocarcinoma, exhibit the ability to differentiate into cells possessing characteristics of small intestinal enterocytes. This makes them a valuable in vitro model for studying iron absorption and bioavailability. Studies using Caco-2 cells have shown that iron transport across cell monolayers is greater from ferric lactoferrin compared to this compound and iron-loaded transferrin. []
Q14: What are the effects of this compound on acute ischemic stroke in rats?
A: In rat models, iron overload prior to induced stroke has been shown to increase infarct size. Interestingly, pretreatment with tempol, a superoxide dismutase mimetic, was able to reverse this effect. This suggests that reactive oxygen species play a significant role in iron-induced damage during stroke. []
A14: The provided research abstracts do not include information regarding resistance mechanisms, cross-resistance, targeted drug delivery, biomarkers, or specific diagnostic applications related to this compound.
Q15: What analytical techniques have been employed to study this compound?
A: Researchers have utilized a variety of techniques to characterize and study this compound. These include:* Electrochemistry: To investigate the redox properties of this compound complexes. []* Electron Spin Resonance (ESR): To study the formation and behavior of free radicals in reactions involving this compound. []* X-ray Diffraction (XRD): To determine the phase composition and crystallinity of this compound and its derivatives. [, ]* Mössbauer Spectroscopy: To investigate the oxidation state, coordination environment, and magnetic properties of iron atoms in this compound complexes. [, ]* Flow Cytometry: To identify and analyze cells treated with this compound. []* Real-time Quantitative Reverse Transcriptase-Polymerase Chain Reaction (RT-qPCR): To quantify gene expression changes in response to this compound treatment. [, ]* Electron Microscopy: To visualize the morphology and structure of this compound nanoparticles and their interactions with cells. [, ]
Disclaimer and Information on In-Vitro Research Products
Please be aware that all articles and product information presented on BenchChem are intended solely for informational purposes. The products available for purchase on BenchChem are specifically designed for in-vitro studies, which are conducted outside of living organisms. In-vitro studies, derived from the Latin term "in glass," involve experiments performed in controlled laboratory settings using cells or tissues. It is important to note that these products are not categorized as medicines or drugs, and they have not received approval from the FDA for the prevention, treatment, or cure of any medical condition, ailment, or disease. We must emphasize that any form of bodily introduction of these products into humans or animals is strictly prohibited by law. It is essential to adhere to these guidelines to ensure compliance with legal and ethical standards in research and experimentation.