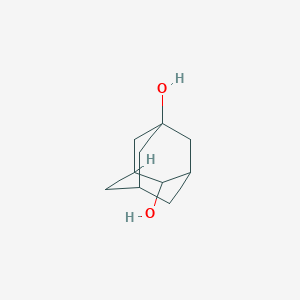
Adamantane-1,4-diol
Overview
Description
Synthesis Analysis
The synthesis of adamantane derivatives involves innovative approaches to introduce functional groups onto the adamantane scaffold, enhancing its reactivity and utility. For example, the one-pot synthesis technique utilizing ethyl 2,4-dioxocyclohexanecarboxylate with specific acrylates via domino Michael reactions and subsequent condensation or aldol-type reactions demonstrates the creative strategies employed to construct adamantane derivatives, including potential pathways for adamantane-1,4-diol (Takagi et al., 2005)(Takagi et al., 2005).
Scientific Research Applications
Adamantane in Clinical Practice
Adamantane derivatives, including adamantane-1,4-diol, have a wide range of applications in various fields of pharmaceuticals. They are key components in drugs used for systemic and topical therapy. These compounds have been proven essential in treating diseases like Alzheimer's, Parkinson's, and diabetes, as well as in antiviral medications. The review by Spilovska et al. (2016) provides comprehensive insights into the mechanisms of action, pharmacokinetics, pharmacodynamics, and clinical trials of adamantane derivatives (Spilovska et al., 2016).
Medicinal Chemistry Applications
Adamantane structures have been significantly utilized in medicinal chemistry. Lamoureux and Artavia (2010) discuss the absorption, distribution, metabolism, excretion (ADME) properties, hydrophobic effects, and ion channel interactions of adamantane derivatives. They highlight how these properties contribute to the development of new drugs (Lamoureux & Artavia, 2010).
Drug Delivery Systems and Surface Recognition
Adamantane-based structures, including this compound, are being explored in the design and synthesis of new drug delivery systems and in surface recognition studies. The research by Štimac et al. (2017) focuses on liposomes, cyclodextrins, and dendrimers that incorporate adamantane derivatives, noting their potential in targeted drug delivery (Štimac et al., 2017).
Soluble Epoxide Hydrolase Inhibition
The effects of adamantane alterations on soluble epoxide hydrolase inhibition potency and metabolic stability are examined by Burmistrov et al. (2018). Their systematic study of adamantyl ureas and diureas contributes valuable data to understand how adamantane modifications can affect drug potency and stability (Burmistrov et al., 2018).
Synthesis of Adamantane Derivatives
Zefirova et al. (2002) report on the synthesis of 1,4-disubstituted adamantanes with amino acid fragments. Their work demonstrates the possibility of creating adamantane derivatives with potential anticancer activity (Zefirova et al., 2002).
Functionalized Adamantanes in Medicinal Therapeutics
Jasys et al. (2000) discuss the synthesis of fluoroadamantane acids and amines, assessing their impact on properties critical for medicinal therapeutics, such as acidity and lipophilicity (Jasys et al., 2000).
Safety and Hazards
Future Directions
The adamantane moiety is widely applied in the design and synthesis of new drug delivery systems and in surface recognition studies . The results reported encourage the development of novel adamantane-based structures and self-assembled supramolecular systems for basic chemical investigations as well as for biomedical application .
Mechanism of Action
Target of Action
Adamantane-1,4-diol, like other adamantane derivatives, is known to interact with various targets. One such target is the Camphor 5-monooxygenase . This enzyme is found in Pseudomonas putida, a bacterium, and plays a crucial role in the metabolism of camphor . .
Mode of Action
The mode of action of adamantane derivatives is complex and can vary depending on the specific derivative and target. For instance, amantadine, an adamantane derivative, is known to block the viral E protein ion channel, leading to impaired viral propagation . It also down-regulates the protease Cathepsin L due to the increase of lysosomal pH, resulting in impaired viral entry and replication
Biochemical Pathways
Adamantane derivatives have been found to affect various biochemical pathways. The synthesis of substituted adamantanes is frequently achieved via carbocation or radical intermediates that have unique stability and reactivity when compared to simple hydrocarbon derivatives . These reactions directly convert diamondoid C–H bonds to C–C bonds, providing a variety of products incorporating diverse functional groups .
Pharmacokinetics
Adamantane and its derivatives are known for their hydrophobic nature, which can influence their absorption, distribution, metabolism, and excretion (adme) properties . The rigid cage structure of adamantane shields adjacent functional groups from metabolic cleavage, thereby augmenting drug stability and distribution in blood plasma .
Result of Action
Adamantane derivatives have been found to disrupt various enzymes, showcasing a wide array of therapeutic activities . These include anti-inflammatory, antiviral, antibacterial, antimicrobial, anticancer, anti-Parkinson, and antidiabetic effects .
Action Environment
The action of this compound, like other adamantane derivatives, can be influenced by environmental factors. For instance, the hydrophobic nature of adamantane allows it to anchor in the lipid bilayer of liposomes, which has promising applications in the field of targeted drug delivery and surface recognition . This suggests that the lipid environment can significantly influence the action, efficacy, and stability of adamantane derivatives.
Biochemical Analysis
Biochemical Properties
Adamantane-1,4-diol plays a significant role in various biochemical reactions. It interacts with a range of enzymes, proteins, and other biomolecules. For instance, it has been observed to interact with cytochrome P450 enzymes, which are crucial for the metabolism of many substances in the body . The hydroxyl groups in this compound can form hydrogen bonds with amino acid residues in enzyme active sites, influencing the enzyme’s activity. Additionally, this compound can act as a substrate for certain oxidoreductases, leading to the formation of oxidized products .
Cellular Effects
This compound affects various types of cells and cellular processes. It has been shown to influence cell signaling pathways, particularly those involving reactive oxygen species (ROS). By modulating ROS levels, this compound can impact gene expression and cellular metabolism . In some cell types, it has been observed to enhance the expression of antioxidant enzymes, thereby protecting cells from oxidative stress . Furthermore, this compound can affect cellular proliferation and apoptosis, making it a compound of interest in cancer research .
Molecular Mechanism
The molecular mechanism of action of this compound involves several key interactions at the molecular level. It can bind to specific biomolecules, such as proteins and nucleic acids, through hydrogen bonding and hydrophobic interactions . This binding can lead to enzyme inhibition or activation, depending on the context. For example, this compound has been shown to inhibit certain proteases by occupying their active sites . Additionally, it can influence gene expression by interacting with transcription factors and other regulatory proteins .
Temporal Effects in Laboratory Settings
In laboratory settings, the effects of this compound can change over time. The compound is relatively stable under standard laboratory conditions, but it can degrade when exposed to extreme pH or high temperatures . Over extended periods, this compound may undergo oxidation, leading to the formation of quinone-like structures . Long-term studies have shown that the compound can have sustained effects on cellular function, including prolonged activation of antioxidant pathways .
Dosage Effects in Animal Models
The effects of this compound vary with different dosages in animal models. At low doses, the compound has been observed to enhance cognitive function and reduce oxidative stress in rodents . At higher doses, it can exhibit toxic effects, such as liver damage and neurotoxicity . There is a threshold beyond which the beneficial effects of this compound are outweighed by its adverse effects . These findings highlight the importance of dosage optimization in potential therapeutic applications.
Metabolic Pathways
This compound is involved in several metabolic pathways. It is primarily metabolized by cytochrome P450 enzymes, leading to the formation of hydroxylated and oxidized metabolites . These metabolites can further participate in conjugation reactions, such as glucuronidation and sulfation, facilitating their excretion from the body . The metabolic flux of this compound can influence the levels of various metabolites, impacting overall metabolic homeostasis .
Transport and Distribution
Within cells and tissues, this compound is transported and distributed through passive diffusion and active transport mechanisms . It can interact with specific transporters, such as organic anion transporters, which facilitate its movement across cellular membranes . The compound tends to accumulate in lipid-rich tissues due to its hydrophobic nature, affecting its localization and bioavailability .
properties
IUPAC Name |
adamantane-1,4-diol | |
---|---|---|
Source | PubChem | |
URL | https://pubchem.ncbi.nlm.nih.gov | |
Description | Data deposited in or computed by PubChem | |
InChI |
InChI=1S/C10H16O2/c11-9-7-1-6-2-8(9)5-10(12,3-6)4-7/h6-9,11-12H,1-5H2 | |
Source | PubChem | |
URL | https://pubchem.ncbi.nlm.nih.gov | |
Description | Data deposited in or computed by PubChem | |
InChI Key |
AUKWRHADVIQZRJ-UHFFFAOYSA-N | |
Source | PubChem | |
URL | https://pubchem.ncbi.nlm.nih.gov | |
Description | Data deposited in or computed by PubChem | |
Canonical SMILES |
C1C2CC3CC(C2)(CC1C3O)O | |
Source | PubChem | |
URL | https://pubchem.ncbi.nlm.nih.gov | |
Description | Data deposited in or computed by PubChem | |
Molecular Formula |
C10H16O2 | |
Source | PubChem | |
URL | https://pubchem.ncbi.nlm.nih.gov | |
Description | Data deposited in or computed by PubChem | |
DSSTOX Substance ID |
DTXSID50401245 | |
Record name | adamantane-1,4-diol | |
Source | EPA DSSTox | |
URL | https://comptox.epa.gov/dashboard/DTXSID50401245 | |
Description | DSSTox provides a high quality public chemistry resource for supporting improved predictive toxicology. | |
Molecular Weight |
168.23 g/mol | |
Source | PubChem | |
URL | https://pubchem.ncbi.nlm.nih.gov | |
Description | Data deposited in or computed by PubChem | |
CAS RN |
20098-16-2 | |
Record name | adamantane-1,4-diol | |
Source | EPA DSSTox | |
URL | https://comptox.epa.gov/dashboard/DTXSID50401245 | |
Description | DSSTox provides a high quality public chemistry resource for supporting improved predictive toxicology. | |
Synthesis routes and methods I
Procedure details
Synthesis routes and methods II
Procedure details
Synthesis routes and methods III
Procedure details
Retrosynthesis Analysis
AI-Powered Synthesis Planning: Our tool employs the Template_relevance Pistachio, Template_relevance Bkms_metabolic, Template_relevance Pistachio_ringbreaker, Template_relevance Reaxys, Template_relevance Reaxys_biocatalysis model, leveraging a vast database of chemical reactions to predict feasible synthetic routes.
One-Step Synthesis Focus: Specifically designed for one-step synthesis, it provides concise and direct routes for your target compounds, streamlining the synthesis process.
Accurate Predictions: Utilizing the extensive PISTACHIO, BKMS_METABOLIC, PISTACHIO_RINGBREAKER, REAXYS, REAXYS_BIOCATALYSIS database, our tool offers high-accuracy predictions, reflecting the latest in chemical research and data.
Strategy Settings
Precursor scoring | Relevance Heuristic |
---|---|
Min. plausibility | 0.01 |
Model | Template_relevance |
Template Set | Pistachio/Bkms_metabolic/Pistachio_ringbreaker/Reaxys/Reaxys_biocatalysis |
Top-N result to add to graph | 6 |
Feasible Synthetic Routes
Q & A
Q1: What is currently known about the pharmacokinetics of adamantane-1,4-diol?
A1: Research indicates that there are significant interspecies differences in the pharmacokinetics of this compound. [, ] This means that the way this metabolite is absorbed, distributed, metabolized, and eliminated from the body varies significantly between species, including rats, rabbits, and humans. This information is crucial for understanding how the effects of kemantane might differ across species.
Q2: Why are interspecies differences in pharmacokinetics important?
A2: Interspecies differences in pharmacokinetics can significantly impact the translation of drug effects from animal models to humans. [, ] A drug or metabolite might be quickly eliminated in one species, leading to low exposure and minimal effects, while accumulating to higher levels in another species, potentially causing toxicity. Therefore, understanding these differences is crucial for extrapolating preclinical data and designing safe and effective human clinical trials.
Q3: Are there any analytical methods available for studying this compound?
A3: While the provided abstracts don't delve into specific methods for this compound analysis, one study mentions developing and validating a GC-FID method for quantifying impurities in kemantane drug substance. [] This suggests that similar gas chromatography techniques, potentially coupled with mass spectrometry, could be employed to detect and quantify this compound in biological samples.
Disclaimer and Information on In-Vitro Research Products
Please be aware that all articles and product information presented on BenchChem are intended solely for informational purposes. The products available for purchase on BenchChem are specifically designed for in-vitro studies, which are conducted outside of living organisms. In-vitro studies, derived from the Latin term "in glass," involve experiments performed in controlled laboratory settings using cells or tissues. It is important to note that these products are not categorized as medicines or drugs, and they have not received approval from the FDA for the prevention, treatment, or cure of any medical condition, ailment, or disease. We must emphasize that any form of bodily introduction of these products into humans or animals is strictly prohibited by law. It is essential to adhere to these guidelines to ensure compliance with legal and ethical standards in research and experimentation.