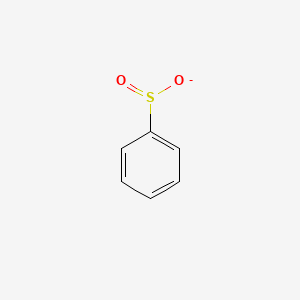
Benzenesulfinate
Overview
Description
Benzenesulfinate (sodium this compound, C₆H₅SO₂Na) is a versatile sulfur-containing nucleophile widely employed in organic synthesis, protein modification, and catalysis. Its reactivity stems from the sulfinate anion (-SO₂⁻), which participates in nucleophilic substitutions, homocoupling reactions, and redox processes. Key applications include:
- Protein cysteine modification: Under acidic conditions, this compound traps nitrosylated cysteine residues via nucleophilic attack, forming stable disulfide adducts (e.g., S-phenylthiosulfonates) .
- Homocoupling reactions: With Fe powder and HCl, sodium this compound yields 1,2-diphenyldisulfane (94% efficiency) .
- Cross-coupling catalysis: It serves as a sulfonylating agent in metal-organic frameworks (e.g., Ni@Bpy-sp2c-COF) for C–S bond formation .
Preparation Methods
Synthetic Routes and Reaction Conditions
-
Phosphorus Pentachloride Method
-
Phosphorus Oxychloride Method
-
Chlorosulfonic Acid Method
Industrial Production Methods
Industrial production of benzenesulfonyl chloride typically involves the chlorination of benzenesulfonic acid or its salts using phosphorus oxychloride or chlorosulfonic acid. These methods are preferred due to their efficiency and scalability .
Chemical Reactions Analysis
Types of Reactions
-
Substitution Reactions
-
Reduction Reactions
-
Oxidation Reactions
Common Reagents and Conditions
Amines: Used in the formation of sulfonamides.
Alcohols: Used in the formation of sulfonate esters.
Zinc Dust: Used in reduction reactions.
Sulfuric Acid: Used in both reduction and oxidation reactions.
Major Products
Sulfonamides: Formed from the reaction with amines.
Sulfonate Esters: Formed from the reaction with alcohols.
Benzenesulfinic Acid: Formed from reduction reactions.
Benzenesulfonic Acid: Formed from oxidation reactions.
Scientific Research Applications
Benzenesulfonyl compounds have a wide range of applications in scientific research:
-
Chemistry
- Used as intermediates in organic synthesis.
- Employed in the preparation of various sulfonamide and sulfonate ester derivatives .
-
Biology
- Used in the study of enzyme inhibition and protein modification.
- Benzenesulfonyl derivatives are used as inhibitors of human neutrophil elastase, which is involved in inflammatory responses .
-
Medicine
-
Industry
- Used in the production of detergents and surfactants.
- Employed in the synthesis of pharmaceuticals and agrochemicals .
Mechanism of Action
The mechanism of action of benzenesulfonyl compounds involves their interaction with specific molecular targets and pathways:
-
Enzyme Inhibition
-
Protein Modification
Comparison with Similar Compounds
Comparative Analysis with Structurally Related Compounds
Sodium Arenesulfinates
Sodium arenesulfinates (e.g., sodium 4-methylbenzenesulfinate) share the general structure Ar-SO₂Na but differ in aryl substituents. Comparative studies reveal:
- Reactivity in homocoupling : Sodium this compound outperforms substituted analogs. For instance, in situ-generated sodium 4-methylthis compound yields only 59% diaryl disulfide under identical Fe/HCl conditions, versus 94% for this compound .
- Electronic effects: Electron-withdrawing para-substituents (e.g., -NO₂) reduce reaction rates in ionic additions to acrylic monomers by stabilizing the sulfinate anion, as shown by a Hammett ρ value of -1.23 .
Table 1: Homocoupling Yields of Sodium Arenesulfinates
Halo-Substituted Benzenesulfinates
Halo-substituted derivatives (e.g., 4-iodothis compound) are critical in Pd-catalyzed cross-couplings. Key distinctions include:
- Synthetic accessibility : Halo-substituted benzenesulfonyl chlorides are commercially available, unlike this compound analogs, which require in situ preparation .
- Catalytic efficiency : In C–S coupling reactions, sodium this compound achieves 94% yield with methyl 4-iodobenzoate, while halo-substituted variants often necessitate higher catalyst loadings .
Methyl this compound
Methyl this compound (C₆H₅SO₂CH₃), an ester derivative, exhibits distinct synthetic pathways and applications:
- Synthesis complexity : Requires a three-step process (reduction of benzenesulfonyl chloride, conversion to sulfinyl chloride, and esterification), contrasting with the straightforward preparation of sodium this compound .
- Reactivity: Less nucleophilic than the sulfinate anion but useful in ozonolysis and radical-mediated transformations .
Sodium Methanesulfinate
Sodium methanesulfinate (CH₃SO₂Na) shares the sulfinate core but lacks aromaticity, leading to:
- Reduced stability : Forms less stable diazosulfone derivatives in colorimetric assays compared to this compound .
- pH-dependent reactivity : Methanesulfinate shows negligible reactivity below pH 4, whereas this compound reacts efficiently across a broader pH range .
Benzenesulfonamides
Benzenesulfonamide derivatives (e.g., TrkA inhibitors) differ in biological activity and synthesis:
- Synthetic routes : Sulfonamides are typically prepared via sulfonyl chloride amidation, avoiding the redox steps required for sulfinates .
- Biological applications : Sulfonamides exhibit anti-cancer activity (e.g., anti-GBM) but lack the catalytic versatility of sulfinates in cross-coupling reactions .
Key Research Findings
- Catalytic superiority : Sodium this compound outperforms substituted arenesulfinates in homocoupling and cross-coupling reactions due to optimal electronic and steric properties .
- Substituent effects: Ortho-substituents decrease ionic addition rates by 3–4× more than para-substituents in acrylic monomer reactions .
- Practical limitations : In situ preparation of substituted sulfinates (e.g., 4-methylthis compound) reduces yields, highlighting the importance of pre-synthesized reagents .
Biological Activity
Benzenesulfinate, a compound derived from benzenesulfonic acid, has garnered attention in recent years for its diverse biological activities. This article reviews the biological effects of this compound, focusing on its anticancer properties, potential for causing dermal irritation, and its role in synthetic organic chemistry.
Anticancer Activity
Recent studies have highlighted the anticancer properties of various benzenesulfonate derivatives, including this compound. A notable research effort demonstrated that certain derivatives possess significant anticancer activity against various cancer cell lines. For instance, a study reported that specific 4-methylbenzenesulfonate derivatives exhibited IC50 values below 0.3 µM against K562 cells, indicating potent cytotoxicity compared to standard chemotherapeutics such as imatinib and CP-31398 .
Table 1: Anticancer Activity of this compound Derivatives
Compound | IC50 (µM) | Selectivity Index |
---|---|---|
BS1 | 0.172 | High |
BS2 | 0.246 | Moderate |
BS3 | 0.173 | High |
BS4 | 0.173 | High |
BS5 | 10.19 | Low |
The selectivity index (SI) indicates the ratio of toxic dose to therapeutic dose, with higher values suggesting safer profiles for normal cells. The study also revealed that this compound derivatives caused cell cycle arrest in the G2/M phase, further underscoring their potential as anticancer agents .
Dermal Irritation Potential
In addition to its therapeutic potentials, the safety profile of this compound has been evaluated through dermal irritation studies . Research involving acute dermal irritation assessments on rabbits indicated that this compound and related sulfonates produced only slight irritation at high concentrations (5000 mg/L), with reactions being reversible within 72 hours . This finding is crucial for assessing the safety of compounds containing this compound when used in consumer products or pharmaceuticals.
Table 2: Dermal Irritation Assessment Results
Concentration (mg/L) | Reaction Severity | Reversibility |
---|---|---|
5000 | Slight Irritation | Yes (within 72 hrs) |
2000 | No Irritation | N/A |
1000 | No Irritation | N/A |
Synthetic Applications
This compound also serves as an important building block in synthetic organic chemistry. It has been utilized in the synthesis of various biologically active compounds, including amino alcohols and sulfonamides that exhibit antioxidant and anti-inflammatory activities . The versatility of this compound in organic reactions is attributed to its ability to act as a nucleophile and participate in radical polymerizations .
Case Study: Synthesis of Antioxidant Compounds
A recent study synthesized a series of benzenesulfonamide derivatives that were evaluated for their antioxidant activity. The compounds showed significant inhibition of oxidative stress markers in vitro, suggesting potential therapeutic applications in oxidative stress-related diseases .
Table 3: Biological Activities of Benzenesulfonamide Derivatives
Compound | Antioxidant Activity (%) at 3h | Antimicrobial Activity (MIC mg/mL) |
---|---|---|
Compound A | 94.69 | 6.72 (E. coli) |
Compound B | 89.66 | 6.63 (S. aureus) |
Compound C | 87.83 | - |
Q & A
Q. Basic: How can benzenesulfinate be synthesized and characterized in laboratory settings?
Methodological Answer:
Sodium this compound (CAS 873-55-2) is commonly synthesized via nucleophilic substitution reactions. For example, sodium this compound can be prepared by reacting benzenesulfonyl chloride with sodium hydroxide under controlled pH conditions . Characterization typically involves nuclear magnetic resonance (NMR) to confirm sulfinate ion structure, infrared (IR) spectroscopy to identify S-O stretching vibrations (~1050 cm⁻¹), and elemental analysis to verify purity (>97%) . Storage should be below +30°C in airtight containers to prevent oxidation .
Q. Basic: What safety protocols are essential when handling this compound derivatives?
Methodological Answer:
this compound derivatives (e.g., benzenesulfonyl chloride) require strict adherence to safety protocols due to their reactivity and toxicity. Personal protective equipment (PPE) must include chemical-resistant gloves (JIS T 8116), safety goggles (JIS T 8147), and lab coats. Inhalation risks necessitate fume hood use, and spills should be neutralized with sodium bicarbonate. Immediate rinsing with water is critical for eye/skin exposure, followed by medical evaluation .
Q. Basic: What analytical techniques are suitable for quantifying this compound in aqueous solutions?
Methodological Answer:
High-performance liquid chromatography (HPLC) with UV detection is widely used. For complex matrices (e.g., electroplating solutions), desalting via solid-phase extraction (C18 columns) minimizes interference. Calibration curves (linearity: R² > 0.993) and spike-recovery tests (91–98%) ensure accuracy. Detection limits for sodium this compound in nickel plating solutions are ~0.1 ppm .
Q. Advanced: How do substituents on the benzene ring influence the reactivity of this compound ions in Michael-type additions?
Methodological Answer:
Electron-withdrawing para-substituents (e.g., -NO₂) decrease ionic addition rates by destabilizing the sulfinate anion, as shown by Hammett correlations (ρ = -1.23 for acrylamide reactions). Ortho-substituents introduce steric hindrance, reducing rates 3–4× more than para-analogues. Kinetic studies (Arrhenius parameters: k = 2.2 × 10⁷ exp(-17.5 kcal/RT)) reveal temperature sensitivity .
Q. Advanced: What catalytic mechanisms enhance this compound reactivity in sulfone formation?
Methodological Answer:
Zinc ions accelerate this compound addition to diacetone acrylamide (DDA) via Lewis acid catalysis, coordinating to sulfinate anions and lowering transition-state energy. However, stoichiometric zinc is required due to deactivation by cyanate byproducts. Rate studies show a 5× increase in k for zinc bis(this compound) compared to sodium salts .
Q. Advanced: How can photopolymerization systems using this compound achieve long-term stability?
Methodological Answer:
Stability in dye-sensitized systems (e.g., Methylene Blue) is achieved by selecting monomers like diacetone acrylamide, which exhibit low dark-reactivity with sulfinate ions. Sterically hindered ortho-substituted benzenesulfinates reduce ionic addition rates while maintaining photopolymer quantum yields (~0.1–1.0). Shelf life is extended by optimizing pH (~7–8) and avoiding acrylic acid monomers, which react rapidly in the dark .
Q. Advanced: What electrochemical methods are used to study this compound redox behavior?
Methodological Answer:
Cyclic voltammetry in dimethyl sulfoxide (DMSO) reveals a two-electron reduction pathway for benzenesulfinic acid at E₁/₂ = -1.2 V (vs. Ag/AgCl). Controlled-potential electrolysis confirms sulfinate-to-sulfonate oxidation, with reaction progress monitored via NMR and IR. Impurities (e.g., sulfonyl chlorides) are excluded by pre-purification using recrystallization .
Q. Advanced: How do steric and electronic effects in acrylic monomers affect this compound addition kinetics?
Methodological Answer:
Methacrylic monomers (e.g., methacrylamide) exhibit slower addition rates than acrylic analogs due to steric hindrance from α-methyl groups. Electron-deficient monomers (e.g., diacetone acrylamide) show 10³× lower rate constants (k ~ 0.01 M⁻¹s⁻¹) compared to hydroxyethyl acrylate (k ~ 10 M⁻¹s⁻¹). Computational modeling (DFT) supports transition-state stabilization by electron-donating monomer groups .
Properties
IUPAC Name |
benzenesulfinate | |
---|---|---|
Details | Computed by Lexichem TK 2.7.0 (PubChem release 2021.10.14) | |
Source | PubChem | |
URL | https://pubchem.ncbi.nlm.nih.gov | |
Description | Data deposited in or computed by PubChem | |
InChI |
InChI=1S/C6H6O2S/c7-9(8)6-4-2-1-3-5-6/h1-5H,(H,7,8)/p-1 | |
Details | Computed by InChI 1.0.6 (PubChem release 2021.10.14) | |
Source | PubChem | |
URL | https://pubchem.ncbi.nlm.nih.gov | |
Description | Data deposited in or computed by PubChem | |
InChI Key |
JEHKKBHWRAXMCH-UHFFFAOYSA-M | |
Details | Computed by InChI 1.0.6 (PubChem release 2021.10.14) | |
Source | PubChem | |
URL | https://pubchem.ncbi.nlm.nih.gov | |
Description | Data deposited in or computed by PubChem | |
Canonical SMILES |
C1=CC=C(C=C1)S(=O)[O-] | |
Details | Computed by OEChem 2.3.0 (PubChem release 2021.10.14) | |
Source | PubChem | |
URL | https://pubchem.ncbi.nlm.nih.gov | |
Description | Data deposited in or computed by PubChem | |
Molecular Formula |
C6H5O2S- | |
Details | Computed by PubChem 2.2 (PubChem release 2021.10.14) | |
Source | PubChem | |
URL | https://pubchem.ncbi.nlm.nih.gov | |
Description | Data deposited in or computed by PubChem | |
DSSTOX Substance ID |
DTXSID50862453 | |
Record name | Benzenesulfinate | |
Source | EPA DSSTox | |
URL | https://comptox.epa.gov/dashboard/DTXSID50862453 | |
Description | DSSTox provides a high quality public chemistry resource for supporting improved predictive toxicology. | |
Molecular Weight |
141.17 g/mol | |
Details | Computed by PubChem 2.2 (PubChem release 2021.10.14) | |
Source | PubChem | |
URL | https://pubchem.ncbi.nlm.nih.gov | |
Description | Data deposited in or computed by PubChem | |
Retrosynthesis Analysis
AI-Powered Synthesis Planning: Our tool employs the Template_relevance Pistachio, Template_relevance Bkms_metabolic, Template_relevance Pistachio_ringbreaker, Template_relevance Reaxys, Template_relevance Reaxys_biocatalysis model, leveraging a vast database of chemical reactions to predict feasible synthetic routes.
One-Step Synthesis Focus: Specifically designed for one-step synthesis, it provides concise and direct routes for your target compounds, streamlining the synthesis process.
Accurate Predictions: Utilizing the extensive PISTACHIO, BKMS_METABOLIC, PISTACHIO_RINGBREAKER, REAXYS, REAXYS_BIOCATALYSIS database, our tool offers high-accuracy predictions, reflecting the latest in chemical research and data.
Strategy Settings
Precursor scoring | Relevance Heuristic |
---|---|
Min. plausibility | 0.01 |
Model | Template_relevance |
Template Set | Pistachio/Bkms_metabolic/Pistachio_ringbreaker/Reaxys/Reaxys_biocatalysis |
Top-N result to add to graph | 6 |
Feasible Synthetic Routes
Disclaimer and Information on In-Vitro Research Products
Please be aware that all articles and product information presented on BenchChem are intended solely for informational purposes. The products available for purchase on BenchChem are specifically designed for in-vitro studies, which are conducted outside of living organisms. In-vitro studies, derived from the Latin term "in glass," involve experiments performed in controlled laboratory settings using cells or tissues. It is important to note that these products are not categorized as medicines or drugs, and they have not received approval from the FDA for the prevention, treatment, or cure of any medical condition, ailment, or disease. We must emphasize that any form of bodily introduction of these products into humans or animals is strictly prohibited by law. It is essential to adhere to these guidelines to ensure compliance with legal and ethical standards in research and experimentation.