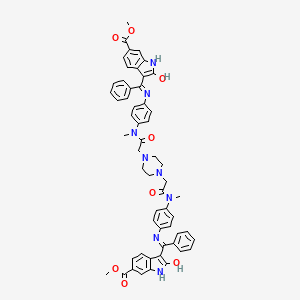
Nintedanib Dimer Impurity
- Click on QUICK INQUIRY to receive a quote from our team of experts.
- With the quality product at a COMPETITIVE price, you can focus more on your research.
Overview
Description
Nintedanib Dimer Impurity is a process-related degradation product or synthetic byproduct formed during the manufacturing of nintedanib, a tyrosine kinase inhibitor used to treat idiopathic pulmonary fibrosis and non-small cell lung cancer. This impurity arises primarily through dimerization reactions under specific conditions, such as elevated temperatures or prolonged storage . SynZeal and other suppliers provide certified reference standards of this impurity for analytical purposes, ensuring compliance with pharmacopeial requirements .
Preparation Methods
Synthetic Routes and Reaction Conditions
The preparation of Nintedanib Dimer Impurity involves the reaction of 3-[methoxyl group (phenyl) methylene]-2-oxoindoline-6-carboxylic acid methyl esters with piperazine in methanol at 60°C. The reaction typically takes 2 to 5 hours to complete . The reaction mixture is then cooled, and the solid product is filtered and washed with methanol to obtain the yellow solid impurity .
Industrial Production Methods
Industrial production methods for this compound are not well-documented in the literature. the general approach involves scaling up the laboratory synthesis process, optimizing reaction conditions, and ensuring consistent quality and yield.
Chemical Reactions Analysis
Types of Reactions
Nintedanib Dimer Impurity can undergo various chemical reactions, including:
Oxidation: The compound can be oxidized under specific conditions to form oxidized derivatives.
Reduction: Reduction reactions can convert the impurity into different reduced forms.
Substitution: The impurity can participate in substitution reactions where functional groups are replaced by other groups.
Common Reagents and Conditions
Common reagents used in these reactions include oxidizing agents like hydrogen peroxide, reducing agents like sodium borohydride, and various catalysts to facilitate substitution reactions. The conditions for these reactions vary depending on the desired product and the specific reaction being performed.
Major Products Formed
The major products formed from these reactions depend on the type of reaction and the reagents used. For example, oxidation may yield oxidized derivatives, while reduction may produce reduced forms of the impurity.
Scientific Research Applications
Quality Control in Pharmaceutical Manufacturing
The Nintedanib dimer impurity serves as a critical reference substance in the quality control processes of pharmaceutical manufacturing. Its quantification is essential for ensuring that the levels of impurities in Nintedanib formulations remain within acceptable limits.
- Detection Methods : High-performance liquid chromatography (HPLC) is commonly employed to analyze the purity of Nintedanib and its impurities. The method typically utilizes octadecylsilane bonded silica as the stationary phase and a mobile phase consisting of potassium dihydrogen phosphate and acetonitrile . The detection wavelength is set at 245 nm to optimize sensitivity.
- Regulatory Standards : Regulatory bodies such as the FDA and EMA require rigorous testing for impurities to ensure patient safety. The content of this compound must be controlled to less than 0.1% during the manufacturing process .
Pharmacological Studies
Research into the pharmacological implications of this compound is crucial for understanding its effects on drug safety and efficacy.
- Toxicology Studies : Studies have been conducted to evaluate the toxicity associated with Nintedanib impurities, including the dimer form. These investigations assess potential adverse effects that may arise from exposure to these impurities during treatment .
- Case Studies : Real-world data has shown that adverse events linked to Nintedanib treatment often correlate with the presence of impurities. For instance, gastrointestinal issues such as diarrhea are prevalent among patients taking Nintedanib, which may be exacerbated by impurities . This highlights the importance of monitoring impurity levels in clinical settings.
Regulatory Compliance and Safety Monitoring
The presence of this compound necessitates strict adherence to regulatory compliance guidelines.
- Risk Management Plans : The European Medicines Agency (EMA) emphasizes the need for comprehensive risk management plans that include monitoring for impurities . This ensures that any potential risks associated with impurities are identified and mitigated effectively.
- Pharmacovigilance : Continuous monitoring of patient outcomes post-marketing is essential for assessing the long-term safety profile of Nintedanib. Reports indicate that adverse events related to Nintedanib can be linked back to its impurities, necessitating ongoing vigilance in pharmacovigilance efforts .
Research and Development
Ongoing research into the synthesis and characterization of this compound contributes to improved understanding and management of this compound.
- Synthesis Techniques : Advances in synthetic methodologies allow for better control over impurity formation during the production of Nintedanib. By optimizing reaction conditions, manufacturers can reduce the formation of undesirable dimers .
- Analytical Chemistry : Continuous development in analytical techniques enhances the ability to detect and quantify impurities accurately. Improved methods can lead to more reliable quality assessments and ensure compliance with regulatory standards.
Mechanism of Action
The mechanism of action of Nintedanib Dimer Impurity is not well-studied. it is likely to interact with similar molecular targets as Nintedanib, such as vascular endothelial growth factor receptors, platelet-derived growth factor receptors, and fibroblast growth factor receptors . These interactions can potentially affect the pathways involved in angiogenesis and fibrosis.
Comparison with Similar Compounds
Structural and Physicochemical Properties
Nintedanib Dimer Impurity is characterized by covalent bonding between two nintedanib molecules, likely through reactive functional groups (e.g., amide or ester linkages).
Key Comparisons :
Compound | CAS Number | Molecular Formula | Molecular Weight (g/mol) | Key Structural Features |
---|---|---|---|---|
This compound | Not explicitly provided | Likely C₆₂H₆₆N₁₀O₈ | ~1,080 | Dimerized nintedanib units |
Nintedanib Acetyl Impurity | 1139458-48-2 | C₂₆H₂₃N₃O₄ | 441.49 | Acetylated piperazine moiety |
Eletriptan Dimer Impurity | Not provided | Not provided | Not provided | Dimerized eletriptan via sulfone hydrolysis |
Ibrutinib Dimer Impurity | Not provided | Not provided | Not provided | Dimerized ibrutinib units |
- Nintedanib Acetyl Impurity (CAS 1139458-48-2) is a structurally distinct impurity formed via acetylation of the parent compound’s piperazine nitrogen. Its lower molecular weight (441.49 g/mol) reflects a single modification rather than dimerization .
- Eletriptan Dimer Impurity () forms during basic hydrolysis of enesulfone intermediates in eletriptan synthesis. Levels are typically reduced to <0.20% during purification, highlighting shared challenges in controlling dimer impurities .
Analytical and Regulatory Challenges
Analytical Methods :
- This compound : Quantified using HPLC with UV detection (237 nm), validated per ICH guidelines to achieve ≤0.1% reporting thresholds .
- Nintedanib Acetyl Impurity : Requires similar HPLC methods but with distinct retention times due to structural differences .
- Eletriptan Dimer Impurity : Characterized via spectroscopic techniques (NMR, MS) and co-injection in HPLC for confirmation .
Regulatory Compliance :
- ICH Q3A/B guidelines require impurities ≥0.10% to be identified and qualified. This compound is controlled to meet these standards, as reflected in SynZeal’s certified reference materials .
Data Tables
Table 1: Comparative Physicochemical Properties
Parameter | This compound | Nintedanib Acetyl Impurity | Eletriptan Dimer Impurity |
---|---|---|---|
Molecular Weight | ~1,080 | 441.49 | Not reported |
CAS Number | Not reported | 1139458-48-2 | Not reported |
Key Functional Groups | Dimer linkage | Acetylated piperazine | Sulfone hydrolysis product |
Table 2: Analytical Methods for Impurity Detection
Method | This compound | Eletriptan Dimer Impurity |
---|---|---|
HPLC Conditions | C18 column; MeCN/H₂O | Not reported |
Detection Wavelength | 237 nm | Not reported |
ICH Compliance | Yes | Yes |
Research Findings
- Dimer impurities are ubiquitous in kinase inhibitor synthesis, necessitating advanced purification and analytical techniques .
- Structural differences between impurities (e.g., dimer vs. acetylated forms) significantly influence detection and quantification strategies .
- Regulatory frameworks ensure impurities remain below thresholds that could compromise drug safety .
Biological Activity
Nintedanib, a multi-kinase inhibitor, primarily targets vascular endothelial growth factor receptors (VEGFR), platelet-derived growth factor receptors (PDGFR), and fibroblast growth factor receptors (FGFR) to impede tumor growth and angiogenesis. The compound also has known impurities, including the Nintedanib Dimer Impurity, which is of particular interest due to its potential biological activity and implications in drug efficacy and safety. This article explores the biological activity of this compound, supported by data tables, case studies, and detailed research findings.
This compound has the following chemical characteristics:
- Molecular Formula: C56H52N8O8
- Molecular Weight: 965.061 g/mol
- Purity: >95% (HPLC)
- SMILES Notation: COC(=O)c1ccc2\C(=C(\Nc3ccc(cc3)N(C)C(=O)CN4CCN(CC(=O)N(C)c5ccc(N\C(=C\6/C(=O)Nc7cc(ccc67)C(=O)OC)\c8ccccc8)cc5)CC4)/c9ccccc9)\C(=O)Nc2c1
These properties indicate that the impurity is a complex organic molecule, likely affecting its interaction with biological systems.
Biological Activity Overview
The biological activity of this compound can be understood through its effects on various cancer models and its interaction with the immune system.
1. Antitumor Activity
Research indicates that Nintedanib itself exhibits antitumor properties by modulating the tumor microenvironment (TME). Although direct cytotoxicity towards certain cancer cells (e.g., B16-F10 melanoma cells) was not observed, Nintedanib was found to enhance antitumor immunity in vivo:
- Study Findings:
The mechanism by which this compound may exert its effects involves several pathways:
- Inhibition of Angiogenesis: By blocking VEGFR, PDGFR, and FGFR signaling pathways, it reduces vascular density in tumors, thereby limiting their growth potential.
- Modulation of Immune Response: It appears to enhance CD8+ T cell activation while inhibiting fibroblast proliferation, which could lead to a less immunosuppressive TME .
Case Studies
Case Study 1: Lung Adenocarcinoma
A study evaluated the effects of Nintedanib in preventing brain metastases in lung adenocarcinoma models. Mice treated with Nintedanib showed a significant reduction in brain metastases volume compared to control groups. This suggests that the dimer impurity may similarly influence metastatic processes through its active components .
Case Study 2: Combination Therapies
In combination with immune checkpoint blockade (ICB), Nintedanib demonstrated enhanced antitumor efficacy. The synergy between Nintedanib and ICB may provide insights into how impurities like the dimer could potentially augment therapeutic outcomes in cancer treatment .
Data Table: Summary of Biological Activities
Q & A
Basic Research Questions
Q. How can researchers develop sensitive analytical methods to detect trace levels of Nintedanib Dimer Impurity in drug substances?
Methodological Answer: Analytical methods must prioritize sensitivity to detect impurities at or below the acceptable intake (AI) limit, guided by regulatory frameworks like ICH Q3A/B. Liquid chromatography-tandem mass spectrometry (LC-MS/MS) or gas chromatography-mass spectrometry (GC-MS/MS) are recommended due to their high specificity and low detection limits (e.g., parts per billion). Method development should follow a "Quality by Design" (QbD) approach, incorporating risk assessments for variables like matrix interference and impurity stability .
Q. What structural characterization techniques are essential for confirming the identity of this compound?
Methodological Answer: Nuclear magnetic resonance (NMR) spectroscopy and high-resolution mass spectrometry (HRMS) are critical for structural elucidation. X-ray crystallography may resolve stereochemical ambiguities, while comparative chromatographic profiling against reference standards (if available) ensures identification accuracy. For novel dimers, stability studies under stress conditions (e.g., thermal, oxidative) can reveal degradation pathways .
Q. What regulatory guidelines govern the establishment of acceptable intake (AI) limits for this compound?
Methodological Answer: AI limits are derived using ICH M7(R2) principles, incorporating compound-specific toxicity data or read-across approaches for structurally related impurities. For nitrosamine-like dimers, the FDA and EMA recommend using the "Threshold of Toxicological Concern" (TTC) when carcinogenicity data are absent. Computational toxicology tools (e.g., QSAR models) and in vitro mutagenicity assays (Ames test) are often required to justify AI limits .
Advanced Research Questions
Q. How should researchers validate analytical methods when contradictory data arise during impurity quantification?
Methodological Answer: Contradictory data may stem from matrix effects, instrument variability, or impurity instability. A tiered validation approach is advised:
- Stage 1: Replicate analyses under controlled conditions (e.g., temperature, pH) to identify variability sources.
- Stage 2: Cross-validate using orthogonal techniques (e.g., LC-MS vs. GC-MS) to confirm impurity identity and concentration.
- Stage 3: Perform robustness testing with spiked placebo matrices to isolate interference factors .
Q. What challenges arise when synthesizing this compound for use as a reference standard?
Methodological Answer: Dimers may form via unintended pathways (e.g., condensation reactions during API synthesis). If direct synthesis is unfeasible, researchers must:
- Characterize the impurity through forced degradation studies.
- Use computational modeling to predict dimer formation mechanisms.
- Justify the absence of the impurity via stability data and reactivity assessments of parent amines, as per EMA and Swissmedic guidelines .
Q. How can Quality by Design (QbD) principles optimize impurity control strategies for this compound?
Methodological Answer: QbD involves defining a "design space" for critical process parameters (CPPs) that influence dimer formation, such as reaction temperature, solvent polarity, and catalyst concentration. Multivariate statistical tools (e.g., partial least squares regression) link CPPs to impurity levels. Real-time monitoring via process analytical technology (PAT) enables dynamic adjustments to suppress dimer generation .
Q. What risk assessment strategies are recommended for this compound in multi-API drug products?
Methodological Answer: Risk assessments must evaluate:
- Drug product compatibility: Interactions between APIs and excipients (e.g., nitrosating agents in capsule shells).
- Process aids: Leachables from manufacturing equipment that may catalyze dimer formation.
- Storage conditions: Humidity and temperature effects on impurity growth. Accelerated stability studies and extractables/leachables (E&L) profiling are critical for holistic risk mitigation .
Q. How can researchers address ultra-low detection limits (e.g., <1 ppm) for genotoxic dimer impurities?
Methodological Answer: Pre-concentration techniques, such as solid-phase extraction (SPE) or liquid-liquid extraction (LLE), enhance sensitivity. For LC-HRMS, isotopic dilution assays using deuterated internal standards improve precision. Collaborative studies with regulatory bodies (e.g., USP’s Nitrosamine Exchange) provide validated non-compendial methods that can be adapted for method validation .
Tables for Quick Reference
Properties
CAS No. |
2410284-90-9 |
---|---|
Molecular Formula |
C56H52N8O8 |
Molecular Weight |
965.1 g/mol |
IUPAC Name |
methyl 2-hydroxy-3-[N-[4-[[2-[4-[2-[4-[[(2-hydroxy-6-methoxycarbonyl-1H-indol-3-yl)-phenylmethylidene]amino]-N-methylanilino]-2-oxoethyl]piperazin-1-yl]acetyl]-methylamino]phenyl]-C-phenylcarbonimidoyl]-1H-indole-6-carboxylate |
InChI |
InChI=1S/C56H52N8O8/c1-61(41-21-17-39(18-22-41)57-51(35-11-7-5-8-12-35)49-43-25-15-37(55(69)71-3)31-45(43)59-53(49)67)47(65)33-63-27-29-64(30-28-63)34-48(66)62(2)42-23-19-40(20-24-42)58-52(36-13-9-6-10-14-36)50-44-26-16-38(56(70)72-4)32-46(44)60-54(50)68/h5-26,31-32,59-60,67-68H,27-30,33-34H2,1-4H3 |
InChI Key |
PLIRRRZAHWLPON-UHFFFAOYSA-N |
Canonical SMILES |
CN(C1=CC=C(C=C1)N=C(C2=CC=CC=C2)C3=C(NC4=C3C=CC(=C4)C(=O)OC)O)C(=O)CN5CCN(CC5)CC(=O)N(C)C6=CC=C(C=C6)N=C(C7=CC=CC=C7)C8=C(NC9=C8C=CC(=C9)C(=O)OC)O |
Origin of Product |
United States |
Disclaimer and Information on In-Vitro Research Products
Please be aware that all articles and product information presented on BenchChem are intended solely for informational purposes. The products available for purchase on BenchChem are specifically designed for in-vitro studies, which are conducted outside of living organisms. In-vitro studies, derived from the Latin term "in glass," involve experiments performed in controlled laboratory settings using cells or tissues. It is important to note that these products are not categorized as medicines or drugs, and they have not received approval from the FDA for the prevention, treatment, or cure of any medical condition, ailment, or disease. We must emphasize that any form of bodily introduction of these products into humans or animals is strictly prohibited by law. It is essential to adhere to these guidelines to ensure compliance with legal and ethical standards in research and experimentation.