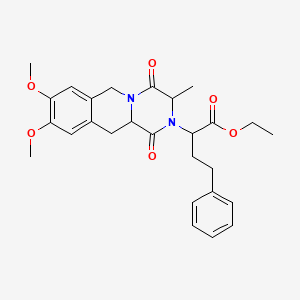
Moexipril Diketopiperazine
- Click on QUICK INQUIRY to receive a quote from our team of experts.
- With the quality product at a COMPETITIVE price, you can focus more on your research.
Overview
Description
Moexipril Diketopiperazine is a cyclization-derived impurity formed during the degradation of Moexipril, an angiotensin-converting enzyme (ACE) inhibitor used to treat hypertension. The diketopiperazine structure arises from intramolecular cyclization of the parent drug, a common degradation pathway observed in ACE inhibitors containing dipeptide-like backbones . While Moexipril itself functions by inhibiting ACE to reduce blood pressure, its diketopiperazine derivative lacks therapeutic activity and is primarily monitored as a stability-related impurity. The formation of this impurity is influenced by environmental factors such as pH, temperature, and formulation strategies, including the use of acid or base addition salts to minimize cyclization .
Preparation Methods
Synthetic Routes and Reaction Conditions
Moexipril Diketopiperazine can be synthesized through various methods, including chemical and enzymatic processes. One common method involves the cyclization of dipeptides under acidic or basic conditions. The reaction typically requires a source of energy, such as heat, to facilitate the formation of the diketopiperazine ring .
Industrial Production Methods
In industrial settings, the production of this compound often involves the use of high-performance liquid chromatography (HPLC) for purification and quality control . The process may include the use of specific reagents and catalysts to optimize yield and purity.
Chemical Reactions Analysis
2.1. Intramolecular Cyclization
Moexipril's degradation to DKP derivatives occurs via intramolecular cyclization, where the carboxyl group of one amino acid residue reacts with the amino group of another, forming a six-membered cyclic amide structure . This reaction is influenced by:
-
Temperature : Elevated temperatures accelerate DKP formation, as seen in enalapril maleate degradation (activation energy: 198 kJ/mol) .
-
pH and solvent : Stability studies of ACE inhibitors like ramipril and cilazapril show accelerated degradation in polar aprotic solvents (e.g., PG, PEG 200) .
2.2. Structural Factors
Moexipril’s tetrahydroisoquinoline core and side-chain extensions influence its reactivity:
-
The 6,7-dimethoxytetrahydroisoquinoline scaffold interacts with the catalytic domain of PDE4 enzymes, but this does not directly contribute to DKP formation .
-
Analogues with shorter N-acyl chains (e.g., compounds 7 and 8 ) show faster reaction kinetics, suggesting steric hindrance in moexipril’s side chain may slow DKP formation .
3.2. Stability and Degradation
Stability studies of ACE inhibitors (including moexipril) reveal:
Vehicle | % Remaining After 5 Days |
---|---|
Water | 85% |
PG | 65% |
PEG 200 | 72% |
DMI | 55% |
(Data adapted from stability studies of similar ACE inhibitors ) |
4.1. Biochemical Potency
Moexipril’s PDE4 inhibitory activity (IC₅₀: 38 μM for PDE4B2) is unrelated to DKP formation but highlights its broader pharmacological profile .
4.2. Kinetics of DKP Formation
-
Isothermal studies : For enalapril maleate (a structural analog), DKP formation follows first-order kinetics above melting points .
-
Model-free analysis : Activation energy calculations using Kissinger–Akahira–Sunose method confirm temperature-dependent degradation pathways .
Implications for Drug Stability and Formulation
DKP formation impacts drug efficacy and shelf-life. For moexipril and similar ACE inhibitors:
Scientific Research Applications
Pharmacological Properties
Moexipril Diketopiperazine exhibits several pharmacological properties that make it a candidate for various therapeutic applications:
- ACE Inhibition : Moexipril itself is primarily known for its ability to inhibit ACE, which plays a critical role in the renin-angiotensin system. This inhibition leads to decreased levels of angiotensin II, resulting in vasodilation and reduced blood pressure .
- PDE4 Inhibition : Recent studies have identified Moexipril as a phosphodiesterase type 4 (PDE4) inhibitor. PDE4 is involved in the hydrolytic degradation of cyclic adenosine monophosphate (cAMP), a crucial second messenger in various cellular processes. Moexipril's ability to inhibit PDE4 may provide anti-inflammatory benefits, which could be leveraged in treating conditions like chronic obstructive pulmonary disease (COPD) and other inflammatory disorders .
Therapeutic Applications
The therapeutic applications of this compound are primarily centered around cardiovascular health and potential anti-inflammatory effects:
- Hypertension Treatment : As an ACE inhibitor, Moexipril is widely used to manage hypertension. It is effective in lowering blood pressure and is often administered in combination with diuretics for enhanced efficacy .
- Potential in COPD : The PDE4 inhibitory activity suggests that Moexipril could be explored as a treatment option for COPD and other inflammatory diseases. Its ability to increase intracellular cAMP levels may lead to improved bronchial dilation and reduced inflammation .
Case Studies and Research Findings
Several studies have documented the effects of Moexipril and its diketopiperazine form:
- Clinical Trials : In controlled clinical trials, Moexipril demonstrated significant antihypertensive effects, with sustained blood pressure reduction over extended periods. The studies indicated that the drug's efficacy was consistent across different demographics, although it was noted to be less effective in black populations compared to non-blacks .
- PDE4 Inhibition Studies : Research utilizing high-throughput screening methods revealed that Moexipril could inhibit PDE4 activity effectively at micromolar concentrations. This finding positions Moexipril as a promising candidate for developing new PDE4 inhibitors with fewer side effects compared to existing options like roflumilast .
Data Table: Summary of Applications
Application Area | Mechanism of Action | Clinical Relevance |
---|---|---|
Hypertension Treatment | ACE inhibition | Reduces blood pressure; widely prescribed |
COPD Management | PDE4 inhibition | Potential anti-inflammatory effects |
Cardiovascular Health | Angiotensin II modulation | Improves vascular function |
Mechanism of Action
Moexipril Diketopiperazine exerts its effects primarily through the inhibition of angiotensin-converting enzyme (ACE). This inhibition prevents the conversion of angiotensin I to angiotensin II, a potent vasoconstrictor, thereby lowering blood pressure . The compound targets ACE and disrupts the renin-angiotensin-aldosterone system (RAAS), leading to vasodilation and reduced blood pressure .
Comparison with Similar Compounds
Structural Comparison
Moexipril Diketopiperazine shares a core six-membered diketopiperazine ring with other ACE inhibitor-derived impurities and natural/synthetic diketopiperazines. Key structural distinctions include:
- Ramipril Diketopiperazine : Contains a complex bicyclic system (cyclopenta[4,5]pyrrolo[1,2-a]pyrazine) and five stereocenters, differentiating it from Moexipril’s simpler diketopiperazine structure .
- Ditryptophenaline: A dimeric diketopiperazine alkaloid with a fused indole-piperazine scaffold, contrasting with Moexipril’s monomeric structure .
Table 1: Structural and Molecular Features
Stability and Degradation Pathways
- This compound : Formation is suppressed via salt stabilization (e.g., hydrochloride salts), a strategy ineffective for Quinapril due to its unique degradation chemistry .
- Quinapril Diketopiperazine : Generates both diketopiperazine and diacid impurities under stress conditions, complicating stabilization efforts compared to Moexipril .
Biological Activity
Moexipril, an angiotensin-converting enzyme (ACE) inhibitor, has gained attention for its potential biological activities beyond its primary use in hypertension management. One of its metabolites, moexipril diketopiperazine, has been studied for various biological effects, particularly its inhibition of phosphodiesterase 4 (PDE4) and its implications in inflammation and other therapeutic areas.
Overview of Moexipril
Moexipril is a prodrug that is converted to its active form, moexiprilat, which exhibits potent ACE inhibitory activity. The compound is administered orally and has a long duration of action, making it suitable for once-daily dosing. Its primary mechanism involves the inhibition of angiotensin II formation, leading to vasodilation and reduced blood pressure. However, recent studies have uncovered additional pharmacological properties that may enhance its therapeutic profile.
PDE4 Inhibition
Recent research has identified moexipril as a PDE4 inhibitor , which plays a crucial role in the hydrolytic degradation of cyclic adenosine monophosphate (cAMP), a significant second messenger involved in various cellular processes. The inhibition of PDE4 can lead to increased intracellular cAMP levels, which have been linked to anti-inflammatory effects and potential neuroprotective properties.
Key Findings:
- Moexipril demonstrated micromolar-range inhibition of three widely expressed PDE4 isoforms: PDE4A4, PDE4B2, and PDE4D5, with the most potent activity observed against PDE4B2 .
- The compound was shown to enhance forskolin-induced increases in intracellular cAMP levels significantly, indicating its potential utility in conditions where cAMP elevation is beneficial .
Molecular Docking Studies
Molecular docking studies have provided insights into how moexipril interacts with the PDE4 catalytic domain. The computational modeling suggests that moexipril binds effectively to the active site of PDE4, which may explain its inhibitory effects on the enzyme's activity. This interaction could pave the way for developing new therapeutic agents targeting PDE4 with improved safety profiles .
Phosphorylation Effects
The elevation of cAMP levels due to PDE4 inhibition by moexipril leads to downstream effects such as the phosphorylation of small heat shock proteins (Hsp20) via protein kinase A pathways. This phosphorylation is critical for various cellular responses, including stress resistance and anti-inflammatory effects .
Pharmacokinetics and Safety Profile
Moexipril exhibits favorable pharmacokinetic properties:
- Bioavailability: Approximately 13% as moexiprilat.
- Half-Life: Moexipril has a half-life of about 1.3 hours, while moexiprilat persists longer with a half-life of approximately 9.8 hours.
- Renal Clearance: The clearance rates indicate that renal function impacts the pharmacokinetics of both moexipril and moexiprilat significantly .
Safety Considerations:
- Long-term studies have shown no evidence of carcinogenicity in animal models at doses up to 14 times the human dose.
- Side effects primarily associated with ACE inhibitors include angioedema; however, these occurrences are rare with moexipril treatment .
Case Studies and Clinical Implications
Several clinical studies have explored the implications of moexipril's biological activities:
- Hypertension Management:
- Inflammatory Conditions:
- Neuroprotection:
Q & A
Basic Research Questions
Q. What are the primary degradation pathways of moexipril leading to diketopiperazine (DKP) formation, and how can these pathways be experimentally validated?
Moexipril undergoes cyclization under acidic and neutral conditions to form DKP as the dominant degradation product, with amide bond hydrolysis occurring at negligible levels . This is consistent with other ACE inhibitors (e.g., ramipril), where intramolecular aminolysis of the dipeptide structure drives DKP formation. Experimental validation involves:
- UPLC-DAD/MS/MS : Monitor degradation kinetics and identify DKP via molecular ions (e.g., m/z 421 for moexipril DKP) and fragmentation patterns (e.g., m/z 375, 347, 319) .
- pH-Varied Forced Degradation : Compare DKP yields under acidic (pH 1–3), neutral (pH 6–7), and alkaline (pH 10) conditions. Alkaline conditions favor ester hydrolysis to dicarboxylic acids (e.g., moexiprilat) rather than DKP .
Condition | Major Product | Key Analytical Markers |
---|---|---|
Acidic | DKP | m/z 421, IR C=O stretches at 1660/1590 cm⁻¹ |
Neutral | DKP | Similar fragmentation to acidic conditions |
Alkaline | Moexiprilat | m/z 411, MS² ions at m/z 365, 206 |
Q. Which spectroscopic methods are most reliable for distinguishing diketopiperazine structures from oxazolones or other cyclic peptides?
- IR Spectroscopy : DKP exhibits two distinct C=O stretching modes (1660 cm⁻¹ and 1590 cm⁻¹) due to amidate resonance differences, whereas oxazolones show a single C=O stretch .
- Hydrogen-Deuterium Exchange (HDX) : DKP structures exchange fewer protons due to rigid ring geometry, while oxazolones show faster exchange rates .
- MS/MS Fragmentation : DKP produces ions like m/z 97 (loss of neutral CO) and m/z 42 (NCO⁻), distinct from oxazolone patterns .
Advanced Research Questions
Q. Why does diketopiperazine formation dominate over amide hydrolysis in moexipril degradation, and how can reaction kinetics resolve this preference?
Cyclization to DKP is entropically and enthalpically favored due to:
- Lower Activation Energy : Intramolecular aminolysis avoids solvent interactions required for hydrolysis .
- Transition-State Stability : The tetrahedral intermediate in DKP formation is stabilized by adjacent carbonyl groups, unlike hydrolysis intermediates . Methodological Approach :
- Kinetic Modeling : Use Arrhenius plots to compare rate constants (k) for cyclization vs. hydrolysis under controlled pH and temperature.
- Computational Studies : Density Functional Theory (DFT) can model energy barriers for competing pathways, as demonstrated in ACE inhibitor degradation studies .
Q. How do stereochemical and substituent effects influence the oxidative functionalization of moexipril-derived diketopiperazines in synthetic applications?
Substituents at C3/C6 positions and stereochemistry dictate regioselectivity in oxidation:
- C11 vs. C15 Hydroxylation : Electron-withdrawing groups (e.g., acetoxy) at C12 increase C15 reactivity, while bulky substituents (e.g., tert-butyl) hinder oxidation at adjacent positions .
- Predictive Modeling : Parameterize permanganate oxidation using substrate-based descriptors (e.g., steric bulk, electron density) to forecast products. For example, bis(pyridine)silver permanganate selectively hydroxylates methylene groups in diketopiperazines with >80% accuracy .
Q. What experimental and computational strategies address contradictions in reported degradation rates of moexipril DKP across studies?
Discrepancies arise from varying buffer systems, catalysts, or detection limits. Resolve by:
- Standardized Protocols : Use ICH Q1A guidelines for forced degradation (e.g., 0.1 M HCl, 40°C) to ensure reproducibility .
- Multivariate Analysis : Apply principal component analysis (PCA) to datasets comparing DKP yields, identifying outliers linked to solvent polarity or ionic strength .
- Machine Learning : Train models on historical ACE inhibitor degradation data to predict moexipril DKP stability under novel conditions .
Q. Can diketopiperazine dimers derived from moexipril enhance pharmacological activity, and what synthetic routes are feasible?
Marine-derived DKP dimers show enhanced receptor binding via bifunctional linkers . For moexipril:
- Biocatalytic Synthesis : Use substrate-permissive diketopiperazine prenyltransferases (e.g., NotF) to dimerize moexipril DKP with terpenoid units .
- Solid-Phase Peptide Synthesis (SPPS) : Avoid DKP formation during synthesis using Fmoc-Pro-Wang resin and low-dielectric solvents .
Q. Data Contradictions & Resolution
- Conflict : Some studies report DKP as the sole degradation product of moexipril, while others detect trace amide hydrolysis by-products .
Resolution : Hydrolysis products (e.g., DP3/DP4) may form transiently but degrade further under prolonged storage. Use real-time stability-indicating assays (e.g., UPLC-DAD at 254 nm) to capture early intermediates .
Properties
Molecular Formula |
C27H32N2O6 |
---|---|
Molecular Weight |
480.6 g/mol |
IUPAC Name |
ethyl 2-(8,9-dimethoxy-3-methyl-1,4-dioxo-3,6,11,11a-tetrahydropyrazino[1,2-b]isoquinolin-2-yl)-4-phenylbutanoate |
InChI |
InChI=1S/C27H32N2O6/c1-5-35-27(32)21(12-11-18-9-7-6-8-10-18)29-17(2)25(30)28-16-20-15-24(34-4)23(33-3)14-19(20)13-22(28)26(29)31/h6-10,14-15,17,21-22H,5,11-13,16H2,1-4H3 |
InChI Key |
UYPPDNXTLSUNRF-UHFFFAOYSA-N |
Canonical SMILES |
CCOC(=O)C(CCC1=CC=CC=C1)N2C(C(=O)N3CC4=CC(=C(C=C4CC3C2=O)OC)OC)C |
Origin of Product |
United States |
Disclaimer and Information on In-Vitro Research Products
Please be aware that all articles and product information presented on BenchChem are intended solely for informational purposes. The products available for purchase on BenchChem are specifically designed for in-vitro studies, which are conducted outside of living organisms. In-vitro studies, derived from the Latin term "in glass," involve experiments performed in controlled laboratory settings using cells or tissues. It is important to note that these products are not categorized as medicines or drugs, and they have not received approval from the FDA for the prevention, treatment, or cure of any medical condition, ailment, or disease. We must emphasize that any form of bodily introduction of these products into humans or animals is strictly prohibited by law. It is essential to adhere to these guidelines to ensure compliance with legal and ethical standards in research and experimentation.