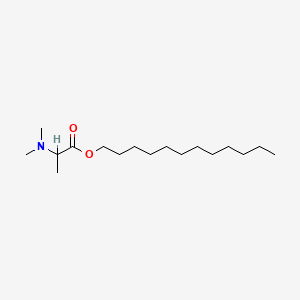
Dodecyl 2-(dimethylamino)propanoate
Overview
Description
Dodecyl 2-(dimethylamino)propanoate is an organic compound with the molecular formula C17H35NO2. It is known for its role as a biodegradable transdermal permeation enhancer, facilitating the delivery of drugs through the skin. This compound is derived from the amino acid alanine and is utilized in various scientific and industrial applications.
Mechanism of Action
Target of Action
Dodecyl 2-(dimethylamino)propanoate, also known as DDAIP, is primarily used as a transdermal permeation enhancer . Its primary target is the skin barrier, specifically the uppermost epidermal layer, the stratum corneum .
Mode of Action
DDAIP interacts with the skin barrier to enhance the permeation of various drugs. It increases the skin’s permeability, allowing drugs to penetrate more effectively .
Pharmacokinetics
It is known to be biodegradable . Its biodegradability is determined using porcine esterase, suggesting that it may be metabolized by esterases in the body .
Result of Action
The primary result of DDAIP’s action is enhanced transdermal drug delivery. It has been shown to significantly increase the skin permeation of several drugs, including theophylline, hydrocortisone, adefovir, and indomethacin . This can lead to improved drug efficacy and patient compliance.
Biochemical Analysis
Biochemical Properties
Dodecyl 2-(dimethylamino)propanoate plays a significant role in biochemical reactions, particularly in enhancing the permeability of the skin to various drugs. This compound interacts with enzymes such as porcine esterase, which facilitates its biodegradation . Additionally, it has been shown to interact with proteins and other biomolecules involved in the skin’s barrier function, thereby increasing the flux of drugs like indomethacin, clonidine, and hydrocortisone . The nature of these interactions involves the disruption of the lipid bilayer of the stratum corneum, allowing for increased drug penetration.
Cellular Effects
This compound has notable effects on various types of cells and cellular processes. It influences cell function by enhancing the permeability of the cell membrane, which can affect cell signaling pathways, gene expression, and cellular metabolism. Studies have shown that this compound can increase the transdermal delivery of drugs without causing significant cytotoxicity . The impact on cell signaling pathways includes the modulation of pathways involved in inflammation and immune response, which can be beneficial for the delivery of anti-inflammatory drugs.
Molecular Mechanism
The molecular mechanism of action of this compound involves its interaction with the lipid components of the cell membrane. This compound binds to the lipid bilayer, causing a disruption that increases membrane fluidity and permeability . Additionally, it can inhibit or activate certain enzymes involved in the metabolism of drugs, thereby enhancing their bioavailability. Changes in gene expression have also been observed, particularly in genes related to skin barrier function and drug metabolism.
Temporal Effects in Laboratory Settings
In laboratory settings, the effects of this compound have been shown to change over time. This compound is relatively stable, but it can undergo degradation in the presence of enzymes like porcine esterase . Long-term studies have indicated that its enhancing effects on drug delivery can be sustained over extended periods without significant loss of activity. The degradation products of this compound may also contribute to its overall efficacy and safety profile.
Dosage Effects in Animal Models
The effects of this compound vary with different dosages in animal models. At low doses, it effectively enhances drug permeability without causing adverse effects . At higher doses, there may be threshold effects that lead to toxicity or adverse reactions. Studies have shown that the optimal dosage range for this compound is crucial for maximizing its benefits while minimizing potential risks.
Metabolic Pathways
This compound is involved in metabolic pathways that include its interaction with enzymes such as porcine esterase . This enzyme catalyzes the hydrolysis of the ester bond in the compound, leading to its biodegradation. The metabolic products of this reaction are further processed by the body’s metabolic machinery, which can affect the overall pharmacokinetics and pharmacodynamics of the drugs being delivered.
Transport and Distribution
Within cells and tissues, this compound is transported and distributed through interactions with transporters and binding proteins . These interactions facilitate its localization to specific areas of the skin where it can exert its permeation-enhancing effects. The compound’s distribution is influenced by factors such as its lipophilicity and the presence of specific binding sites on cell membranes.
Subcellular Localization
The subcellular localization of this compound is primarily within the lipid bilayers of cell membranes . This localization is critical for its activity as a permeation enhancer, as it allows the compound to interact directly with the lipid components of the membrane. Targeting signals and post-translational modifications may also play a role in directing the compound to specific cellular compartments, further enhancing its efficacy.
Preparation Methods
Synthetic Routes and Reaction Conditions: Dodecyl 2-(dimethylamino)propanoate can be synthesized through a reaction involving dodecyl 2-bromopropionate and dimethylamine. The process typically involves the following steps:
Formation of Dodecyl 2-bromopropionate: This intermediate is prepared by reacting n-dodecanol with 2-bromopropionyl halogenide.
Nucleophilic Substitution: The dodecyl 2-bromopropionate is then reacted with dimethylamine to yield this compound.
Industrial Production Methods: In industrial settings, the production of this compound follows similar synthetic routes but on a larger scale. The reaction conditions are optimized to ensure high yield and purity, often involving controlled temperatures and the use of catalysts to enhance reaction efficiency.
Chemical Reactions Analysis
Types of Reactions: Dodecyl 2-(dimethylamino)propanoate undergoes various chemical reactions, including:
Substitution Reactions: The compound can participate in nucleophilic substitution reactions due to the presence of the dimethylamino group.
Common Reagents and Conditions:
Nucleophilic Substitution: Dimethylamine is commonly used as a nucleophile in the synthesis of this compound.
Hydrolysis: Acidic or basic conditions facilitate the hydrolysis of the compound.
Major Products Formed:
Scientific Research Applications
Dodecyl 2-(dimethylamino)propanoate has a wide range of applications in scientific research, including:
Chemistry: It is used as a transdermal permeation enhancer, improving the delivery of various drugs through the skin.
Biology: The compound is studied for its ability to enhance the absorption of biologically active molecules.
Comparison with Similar Compounds
Dodecyl 6-(dimethylamino)hexanoate: Another transdermal permeation enhancer with a longer linking chain, which has shown higher potency in some studies.
Azone: A well-known skin penetration enhancer used for comparison in research studies.
Lauryl Alcohol: A possible decomposition product of dodecyl 2-(dimethylamino)propanoate, used as a standard enhancer in studies.
Uniqueness: this compound is unique due to its balance of effectiveness and biodegradability. It provides significant enhancement of drug permeation while being rapidly metabolized, minimizing potential toxicity .
Properties
IUPAC Name |
dodecyl 2-(dimethylamino)propanoate | |
---|---|---|
Source | PubChem | |
URL | https://pubchem.ncbi.nlm.nih.gov | |
Description | Data deposited in or computed by PubChem | |
InChI |
InChI=1S/C17H35NO2/c1-5-6-7-8-9-10-11-12-13-14-15-20-17(19)16(2)18(3)4/h16H,5-15H2,1-4H3 | |
Source | PubChem | |
URL | https://pubchem.ncbi.nlm.nih.gov | |
Description | Data deposited in or computed by PubChem | |
InChI Key |
HSMMSDWNEJLVRY-UHFFFAOYSA-N | |
Source | PubChem | |
URL | https://pubchem.ncbi.nlm.nih.gov | |
Description | Data deposited in or computed by PubChem | |
Canonical SMILES |
CCCCCCCCCCCCOC(=O)C(C)N(C)C | |
Source | PubChem | |
URL | https://pubchem.ncbi.nlm.nih.gov | |
Description | Data deposited in or computed by PubChem | |
Molecular Formula |
C17H35NO2 | |
Source | PubChem | |
URL | https://pubchem.ncbi.nlm.nih.gov | |
Description | Data deposited in or computed by PubChem | |
DSSTOX Substance ID |
DTXSID70933573 | |
Record name | Dodecyl N,N-dimethylalaninate | |
Source | EPA DSSTox | |
URL | https://comptox.epa.gov/dashboard/DTXSID70933573 | |
Description | DSSTox provides a high quality public chemistry resource for supporting improved predictive toxicology. | |
Molecular Weight |
285.5 g/mol | |
Source | PubChem | |
URL | https://pubchem.ncbi.nlm.nih.gov | |
Description | Data deposited in or computed by PubChem | |
CAS No. |
224297-43-2, 149196-89-4 | |
Record name | Dodecyl 2-(N,N-dimethylamino)propionate | |
Source | CAS Common Chemistry | |
URL | https://commonchemistry.cas.org/detail?cas_rn=224297-43-2 | |
Description | CAS Common Chemistry is an open community resource for accessing chemical information. Nearly 500,000 chemical substances from CAS REGISTRY cover areas of community interest, including common and frequently regulated chemicals, and those relevant to high school and undergraduate chemistry classes. This chemical information, curated by our expert scientists, is provided in alignment with our mission as a division of the American Chemical Society. | |
Explanation | The data from CAS Common Chemistry is provided under a CC-BY-NC 4.0 license, unless otherwise stated. | |
Record name | Dodecyl N,N-dimethylalaninate | |
Source | EPA DSSTox | |
URL | https://comptox.epa.gov/dashboard/DTXSID70933573 | |
Description | DSSTox provides a high quality public chemistry resource for supporting improved predictive toxicology. | |
Synthesis routes and methods I
Procedure details
Synthesis routes and methods II
Procedure details
Retrosynthesis Analysis
AI-Powered Synthesis Planning: Our tool employs the Template_relevance Pistachio, Template_relevance Bkms_metabolic, Template_relevance Pistachio_ringbreaker, Template_relevance Reaxys, Template_relevance Reaxys_biocatalysis model, leveraging a vast database of chemical reactions to predict feasible synthetic routes.
One-Step Synthesis Focus: Specifically designed for one-step synthesis, it provides concise and direct routes for your target compounds, streamlining the synthesis process.
Accurate Predictions: Utilizing the extensive PISTACHIO, BKMS_METABOLIC, PISTACHIO_RINGBREAKER, REAXYS, REAXYS_BIOCATALYSIS database, our tool offers high-accuracy predictions, reflecting the latest in chemical research and data.
Strategy Settings
Precursor scoring | Relevance Heuristic |
---|---|
Min. plausibility | 0.01 |
Model | Template_relevance |
Template Set | Pistachio/Bkms_metabolic/Pistachio_ringbreaker/Reaxys/Reaxys_biocatalysis |
Top-N result to add to graph | 6 |
Feasible Synthetic Routes
Q1: What structural features of Dodecyl 2-(dimethylamino)propanoate contribute to its effectiveness as a transdermal permeation enhancer?
A1: While the provided abstract [] does not specifically address this compound, it highlights the importance of linking chain length, chirality, and polyfluorination in dimethylamino acid esters for transdermal permeation enhancement. Longer alkyl chains, like the dodecyl group in this compound, can interact with the lipid bilayer of the skin, potentially disrupting its organization and enhancing drug permeation. The chirality of the molecule can influence its interaction with chiral components of the skin, potentially affecting its efficacy. Polyfluorination, although not present in this compound, is mentioned as a modification that can impact permeation enhancement, likely due to altered lipophilicity and hydrogen bonding capacity. Further research is needed to elucidate the specific contributions of each structural feature in this compound's efficacy as a permeation enhancer.
Disclaimer and Information on In-Vitro Research Products
Please be aware that all articles and product information presented on BenchChem are intended solely for informational purposes. The products available for purchase on BenchChem are specifically designed for in-vitro studies, which are conducted outside of living organisms. In-vitro studies, derived from the Latin term "in glass," involve experiments performed in controlled laboratory settings using cells or tissues. It is important to note that these products are not categorized as medicines or drugs, and they have not received approval from the FDA for the prevention, treatment, or cure of any medical condition, ailment, or disease. We must emphasize that any form of bodily introduction of these products into humans or animals is strictly prohibited by law. It is essential to adhere to these guidelines to ensure compliance with legal and ethical standards in research and experimentation.