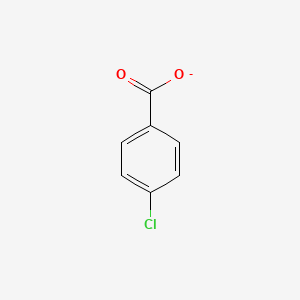
4-Chlorobenzoate
Overview
Description
4-Chlorobenzoate (4-CBA, C₇H₄ClO₂⁻) is a chlorinated aromatic compound with a chlorine substituent at the para position of the benzoate ring. It serves as a critical intermediate in organic synthesis, pharmaceutical production, and microbial degradation pathways. The electron-withdrawing chlorine atom significantly influences its reactivity, solubility, and interactions with biological systems. 4-CBA is a substrate for specialized enzymes like this compound:CoA ligase (CBL), which activates it for subsequent dehalogenation in bioremediation pathways .
Scientific Research Applications
Bioremediation
Role in Degradation Pathways
4-Chlorobenzoate is a significant intermediate in the microbial degradation of polychlorinated biphenyls (PCBs). Certain bacterial strains, such as Alcaligenes sp. strain ALP83, utilize this compound as a substrate to metabolize PCBs into less harmful substances. The degradation pathway involves the conversion of this compound into 4-hydroxybenzoate, which can then be further degraded to carbon dioxide and other benign products . This bioremediation process is crucial for detoxifying environments contaminated with chlorinated aromatic compounds.
Enzymatic Mechanisms
The enzymatic degradation of this compound is facilitated by specific enzymes like this compound:Coenzyme A ligase (CBL). This enzyme catalyzes the thioesterification of this compound, enhancing its reactivity and enabling further breakdown through microbial pathways. Mutations in the CBL enzyme have been engineered to improve its efficiency in catalyzing reactions with various chlorinated substrates, showcasing the potential for biotechnological applications in environmental cleanup .
Chemical Synthesis
Synthesis of Derivatives
this compound serves as a versatile building block in organic synthesis. It can be transformed into various derivatives that possess biological activity. For instance, acyl thiourea derivatives synthesized from this compound have shown promising antibacterial and antifungal properties . These derivatives are characterized through spectroscopic methods and demonstrate enhanced biological activity compared to their parent compounds.
Medicinal Chemistry
In medicinal chemistry, compounds derived from this compound have been explored for their potential therapeutic effects. Research has indicated that certain derivatives exhibit significant inhibitory activity against enzymes like calf intestinal alkaline phosphatase, highlighting their potential use as pharmacological agents . The structural modification of this compound allows for the development of new drugs targeting various diseases.
Environmental Monitoring
Indicator of Pollution
Due to its stability and persistence in the environment, this compound is often used as an indicator compound for monitoring chlorinated pollutants. Its presence in soil and water samples can signal contamination from industrial activities involving chlorinated hydrocarbons. Analytical techniques such as gas chromatography-mass spectrometry (GC-MS) are employed to detect and quantify this compound levels in environmental samples .
Data Tables
Application Area | Description | Example Organisms/Compounds |
---|---|---|
Bioremediation | Degradation of PCBs using microbial pathways | Alcaligenes sp. strain ALP83 |
Chemical Synthesis | Synthesis of biologically active derivatives | Acyl thiourea derivatives |
Environmental Monitoring | Indicator for chlorinated pollutant presence | Soil and water analysis |
Case Study 1: PCB Degradation
A study demonstrated that Alcaligenes sp. strain ALP83 effectively degrades PCBs through a pathway involving this compound. The research highlighted how this strain converts chlorinated biphenyls into less toxic metabolites using enzymatic processes that include CBL activity .
Case Study 2: Synthesis of Antibacterial Agents
Research focused on synthesizing acyl thiourea derivatives from this compound revealed significant antibacterial properties against various pathogens. The study characterized these compounds using nuclear magnetic resonance (NMR) spectroscopy and evaluated their efficacy through inhibition assays .
Chemical Reactions Analysis
Hydrolysis
The ester bond in 4-chlorobenzoate derivatives can undergo hydrolysis in the presence of an acid or base, yielding 4-chlorobenzoic acid and the corresponding alcohol.
Reduction
This compound can be reduced to form the corresponding alcohol. Reducing agents such as lithium aluminum hydride (LiAlH4) or sodium borohydride (NaBH4) can be used.
Substitution
The chlorine atom on the benzene ring can undergo nucleophilic substitution reactions. Nucleophiles such as amines or thiols can be used under appropriate conditions to substitute the chlorine atom.
This compound-CoA Ligase Catalyzed Reactions
This compound—CoA ligase (EC 6.2.1.33) is an enzyme that catalyzes the chemical reaction :
This enzyme belongs to the family of ligases, specifically those forming carbon-sulfur bonds as acid-thiol ligases. The systematic name of this enzyme class is this compound: CoA ligase. This enzyme participates in 2,4-dichlorobenzoate degradation and employs magnesium as a cofactor .
The mechanism of this compound: CoA ligase (CBL) catalysis involves two partial reactions. In conformation 1, CBL catalyzes the adenylation of this compound (4-CB) with ATP. In conformation 2, CBL catalyzes the formation of 4-CB-CoA from 4-CB-AMP and CoA .
Wild-type CBL is activated by Mg+2, and its kinetic mechanism supports the prediction that PP i dissociation must precede the switch from conformation 1 to conformation 2, and therefore CoA binding .
Suzuki-Miyaura Coupling Reaction
Methyl this compound undergoes Suzuki-Miyaura coupling reaction with phenylboronic acid in the presence of a bis-carbene palladium complex catalyst to yield methyl-(4-phenyl)-benzoate .
Reaction with Organotin(IV) Chlorides
Dibutyltin(IV) di-4-chlorobenzoate, diphenyltin(IV) di-4-chlorobenzoate, and triphenyltin(IV) this compound can be synthesized from their respective chlorides . For instance, dibutyltin(IV) di-4-chlorobenzoate is prepared according to the following reaction scheme :
Table 1: Microanalytical data of organotin(IV) compounds synthesized
Compound | Carbon (Calculated) | Hydrogen (Calculated) |
---|---|---|
$$(n-C4H9)2Sn(4 –C6H4(Cl)COO)2] | 48.2 (48.5) | 4.8 (4.8) |
$$(C6H5)2Sn(4 –C6H4(Cl)COO)2] | 53.2 (53.4) | 3.3 (3.1) |
$$(C6H5)3Sn(4 –C6H4(Cl)COO)] | 57.5 (57.6) | 3.8 (3.7) |
Table 2: Selected IR spectral data of the compounds synthesized (cm-1)
Compound | Sn-O | Sn-O-C | Sn-Bu | C=O | C-H aliphatic | Phenyl |
---|---|---|---|---|---|---|
$$(n-C4H9)2Sn(4 –C6H4(Cl)COO)2] | 434.6 | 1029.2 | 674.9 | 1419.2, 1558.2 | 2954 – 2860 | – |
$$(C6H5)2Sn(4 –C6H4(Cl)COO)2] | 591.1 | 1290.4 | – | 1596.7, 1690.3 | – | 1490.5; 725.7 |
$$(C6H5)3Sn(4 –C6H4(Cl)COO)] | 765.50 | 1243.3 | – | 1558.3, 1631.6 | – | 1428.4; 729.4 |
Q & A
Q. What are the standard analytical methods for quantifying 4-chlorobenzoate in environmental or biological samples?
Answer:
Quantification typically involves high-performance liquid chromatography (HPLC) paired with UV-Vis detection, calibrated using standard curves of purified this compound. For complex matrices (e.g., soil or microbial lysates), solid-phase extraction (SPE) or liquid-liquid extraction is recommended to isolate the compound. In biodegradation studies, fed-batch techniques with acclimated biomass can track degradation rates via substrate depletion assays . For enzyme studies, the Bradford method (protein-dye binding) is critical for normalizing enzyme activity to total protein concentration during purification .
Q. What is the role of the fcb gene cluster in this compound degradation, and how can its expression be experimentally validated?
Answer:
The fcb cluster (fcbB-fcbA-fcbT1-T2-T3-fcbC) in Comamonas sp. DJ-12 mediates hydrolytic dehalogenation of this compound. To validate expression:
- Induction assays: Grow bacteria with 4-chloro-, 4-bromo-, or 4-iodobenzoate as sole carbon sources and monitor gene activation via RT-qPCR .
- Enzyme activity assays: Measure this compound:CoA ligase (CBL) activity in cell lysates using ATP-dependent CoA thioester formation, detected by HPLC or spectrophotometric assays (e.g., NADH-coupled systems) .
- Knockout mutants: Generate fcb deletion strains and compare degradation efficiency with wild-type strains .
Q. How can researchers resolve contradictions in reported microbial degradation efficiencies of this compound?
Answer:
Contradictions often arise from strain-specific enzyme kinetics or experimental conditions. Address this by:
- Kinetic profiling: Compare kcat and Km values of CBL across bacterial strains (e.g., Pseudomonas vs. Comamonas) to identify substrate affinity variations .
- Structural analysis: Use X-ray crystallography or docking studies to assess active-site residues (e.g., Lys107 in Acidovorax sp. KKS102’s dehalogenase) that influence substrate specificity .
- Environmental factors: Replicate experiments under varying pH, temperature, or co-substrate conditions to test robustness .
Q. What experimental strategies are used to study conformational dynamics in this compound:CoA ligase (CBL)?
Answer:
CBL undergoes a 140° domain rotation between its adenylation (ATP-dependent) and thioesterification (CoA-dependent) states. Key approaches include:
- Crystallography: Solve structures of CBL bound to intermediates like 4-chlorobenzoyl-adenylate (4-CB-AMP) and 4-chlorophenacyl-CoA (4-CP-CoA) to capture conformational states .
- Transient kinetics: Use stopped-flow assays to measure single-turnover rates for adenylation (k = 300 s<sup>-1</sup>) and thioesterification (k = 120 s<sup>-1</sup>), identifying rate-limiting steps (e.g., product dissociation) .
- Site-directed mutagenesis: Target residues involved in domain alternation (e.g., hinge regions) and assess activity loss via steady-state kinetics .
Q. How should researchers design experiments to investigate anaerobic degradation pathways of this compound?
Answer:
Anaerobic pathways often involve reductive dechlorination or CoA-mediated steps. Experimental design considerations:
- Sediment microcosms: Use freshwater sediment slurries amended with this compound and monitor metabolite formation (e.g., 4-hydroxybenzoate) via GC-MS .
- Isotope tracing: Employ <sup>13</sup>C-labeled this compound to track carbon flow and identify intermediates .
- Gene expression profiling: Apply metatranscriptomics to identify upregulated genes (e.g., fcb homologs) in adapted microbial communities .
Q. What methodologies are recommended for determining the rate-limiting step in this compound:CoA ligase catalysis?
Answer:
- Pre-steady-state kinetics: Use rapid-quench or stopped-flow methods to measure burst phases of 4-CB-AMP formation, revealing if adenylation (Partial Reaction 1) or thioesterification (Partial Reaction 2) is slower .
- Mg<sup>2+</sup> dependence assays: Test activation of CBL by Mg<sup>2+</sup> (12–75-fold activity increase), which influences PPi release and domain alternation .
- Product inhibition studies: Add AMP or PPi to determine if product dissociation limits turnover .
Q. How can structural bioinformatics tools aid in engineering this compound-degrading enzymes for expanded substrate ranges?
Answer:
- Homology modeling: Generate 3D models of CBL using templates like anthranilate-CoA ligase (PDB: 3D8T) to predict substrate-binding pockets .
- Docking simulations: Screen virtual libraries of halogenated benzoates against CBL’s active site to prioritize substrates (e.g., 4-bromo- or 4-iodobenzoate) .
- Rational mutagenesis: Redesign residues (e.g., Phe305 in CBL) to accommodate bulkier substituents, followed by kinetic validation .
Comparison with Similar Compounds
Comparative Analysis with Similar Compounds
Reactivity in Chemical Reactions
The chlorine substituent enhances 4-CBA’s reactivity compared to non-chlorinated analogues. For example:
- Vinyl 4-Chlorobenzoate vs. Vinyl Benzoate : Vinyl this compound exhibits higher reactivity in amidation reactions due to the electron-withdrawing effect of the chlorine atom, which facilitates nucleophilic attack. This contrasts with vinyl benzoate, which requires harsher conditions for similar transformations .
- Esterification Efficiency : Methyl this compound is synthesized from 4-CBA via acid-catalyzed esterification (H₂SO₄/MeOH, 88% yield) , while 2-oxo-2H-chromen-7-yl this compound is prepared using 4-chlorobenzoyl chloride and triethylamine in dichloromethane (88% yield) . Both methods highlight the versatility of 4-CBA derivatives in forming esters under mild conditions.
Enzymatic Interactions and Substrate Specificity
4-CBA’s degradation in bacteria involves specialized enzymes with distinct substrate preferences:
- This compound:CoA Ligase (CBL) : Native CBL selectively activates 4-CBA via adenylation and thioesterification. Rational mutagenesis (e.g., I303A/G mutations) expanded its substrate range to include 3,4-dichlorobenzoate, demonstrating that steric hindrance at the meta position limits native enzyme activity .
- Dehalogenases : Pseudomonas sp. CBS3 dehalogenates 4-CBA to 4-hydroxybenzoate, while Alcaligenes denitrificans NTB-1 processes 2,4-dichlorobenzoate via reductive dechlorination to 4-CBA before dehalogenation . This highlights positional sensitivity in enzymatic pathways.
Table 2: Kinetic Parameters of CBL with Chlorobenzoate Derivatives
Microbial Degradation Pathways
Microorganisms employ divergent strategies for chlorobenzoates:
- 4-CBA : Degraded via hydrolytic dehalogenation to 4-hydroxybenzoate, followed by ring cleavage (e.g., Pseudomonas sp. S-47) .
- 2,4-Dichlorobenzoate : Undergoes reductive dechlorination to 4-CBA before dehalogenation in Alcaligenes denitrificans NTB-1 .
- Meta-Chlorinated Analogues : Poorly metabolized by strains like Arthrobacter sp. TM-1, which exclusively degrades 4-CBA .
Physical and Chemical Properties
The chlorine substituent alters physical properties compared to non-chlorinated benzoates:
Table 3: Physical Properties of 4-CBA and Derivatives
Compound | Melting Point (°C) | Boiling Point (°C) | Density (g/cm³) | Reference |
---|---|---|---|---|
4-Chlorobenzoic Acid | 238–241 | – | – | |
Ethyl this compound | 71–73 | 237–239 | 1.187 | |
Benzoic Acid | 122–125 | 249 | 1.265 | – |
Structural and Spectroscopic Features
- Molecular Geometry : X-ray studies of 2-chloro-6-fluorophenyl this compound reveal bond lengths and angles similar to dichlorinated analogues, indicating minimal structural distortion from additional substituents .
- Spectroscopic Signatures : The C–Cl stretch in 4-CBA derivatives appears at ~744 cm⁻¹ in IR spectra, distinct from C–O–C stretches (~1231 cm⁻¹) .
Properties
Molecular Formula |
C7H4ClO2- |
---|---|
Molecular Weight |
155.56 g/mol |
IUPAC Name |
4-chlorobenzoate |
InChI |
InChI=1S/C7H5ClO2/c8-6-3-1-5(2-4-6)7(9)10/h1-4H,(H,9,10)/p-1 |
InChI Key |
XRHGYUZYPHTUJZ-UHFFFAOYSA-M |
SMILES |
C1=CC(=CC=C1C(=O)[O-])Cl |
Canonical SMILES |
C1=CC(=CC=C1C(=O)[O-])Cl |
Synonyms |
4-chlorobenzoate 4-chlorobenzoic acid 4-chlorobenzoic acid, calcium(2:1) salt 4-chlorobenzoic acid, mercury(+1) salt 4-chlorobenzoic acid, mercury(+2)(2:1) salt 4-chlorobenzoic acid, sodium salt 4-chlorobenzoic acid, sodium salt, 11C-labeled para-chlorobenzoic acid |
Origin of Product |
United States |
Synthesis routes and methods
Procedure details
Retrosynthesis Analysis
AI-Powered Synthesis Planning: Our tool employs the Template_relevance Pistachio, Template_relevance Bkms_metabolic, Template_relevance Pistachio_ringbreaker, Template_relevance Reaxys, Template_relevance Reaxys_biocatalysis model, leveraging a vast database of chemical reactions to predict feasible synthetic routes.
One-Step Synthesis Focus: Specifically designed for one-step synthesis, it provides concise and direct routes for your target compounds, streamlining the synthesis process.
Accurate Predictions: Utilizing the extensive PISTACHIO, BKMS_METABOLIC, PISTACHIO_RINGBREAKER, REAXYS, REAXYS_BIOCATALYSIS database, our tool offers high-accuracy predictions, reflecting the latest in chemical research and data.
Strategy Settings
Precursor scoring | Relevance Heuristic |
---|---|
Min. plausibility | 0.01 |
Model | Template_relevance |
Template Set | Pistachio/Bkms_metabolic/Pistachio_ringbreaker/Reaxys/Reaxys_biocatalysis |
Top-N result to add to graph | 6 |
Feasible Synthetic Routes
Disclaimer and Information on In-Vitro Research Products
Please be aware that all articles and product information presented on BenchChem are intended solely for informational purposes. The products available for purchase on BenchChem are specifically designed for in-vitro studies, which are conducted outside of living organisms. In-vitro studies, derived from the Latin term "in glass," involve experiments performed in controlled laboratory settings using cells or tissues. It is important to note that these products are not categorized as medicines or drugs, and they have not received approval from the FDA for the prevention, treatment, or cure of any medical condition, ailment, or disease. We must emphasize that any form of bodily introduction of these products into humans or animals is strictly prohibited by law. It is essential to adhere to these guidelines to ensure compliance with legal and ethical standards in research and experimentation.