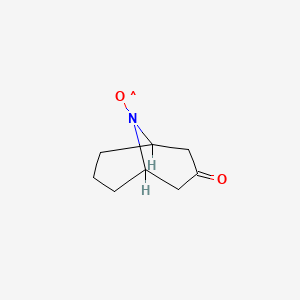
KetoABNO
Overview
Description
Keto-ABNO, also known as 9-azabicyclo[3.3.1]nonan-3-one N-oxyl, is a yellow crystalline solid used primarily as an oxidation catalyst. It is known for its efficiency in catalytic aerobic oxidation and protein modification. Keto-ABNO is bench-stable for several months and soluble in various organic solvents .
Mechanism of Action
Target of Action
KetoABNO, also known as 9-Azabicyclo[3.3.1]nonan-3-one N-oxyl, is primarily used as a catalyst in the oxidation of secondary alcohols . The primary target of this compound is the secondary alcohol substrates, including unactivated aliphatic substrates .
Mode of Action
This compound interacts with its targets (secondary alcohols) through a process known as aerobic oxidation . In this process, this compound, in combination with copper (I), facilitates the oxidation of secondary alcohols to corresponding ketones . This reaction is highly effective and can be performed under ambient conditions .
Biochemical Pathways
It’s known that this compound plays a crucial role in the oxidation process of secondary alcohols . This process is part of the broader metabolic pathways involving the breakdown and synthesis of organic molecules in the body.
Result of Action
The primary result of this compound’s action is the efficient oxidation of secondary alcohols to corresponding ketones . This transformation is particularly significant as it includes unactivated aliphatic substrates, which are typically challenging to oxidize .
Action Environment
The efficacy and stability of this compound are influenced by various environmental factors. For instance, the pressure and gas composition can affect the performance of the this compound-copper (I) catalyst system . Furthermore, this compound is a bench-top stable solid, suggesting that it can maintain its stability under standard laboratory conditions .
Biochemical Analysis
Biochemical Properties
KetoABNO plays a crucial role in biochemical reactions, particularly in the oxidation of alcohols and amines. It acts as an oxidant, facilitating the conversion of alcohols to aldehydes and ketones, and amines to imines . The compound interacts with enzymes such as alcohol dehydrogenase and amine oxidase, enhancing their catalytic activity. These interactions are primarily based on the redox properties of this compound, which allow it to accept and donate electrons during the reaction process .
Cellular Effects
This compound has significant effects on various types of cells and cellular processes. It influences cell function by modulating cell signaling pathways, gene expression, and cellular metabolism. For instance, this compound can induce oxidative stress in cells, leading to the activation of stress response pathways and changes in gene expression . This compound also affects cellular metabolism by altering the redox state of cells, which can impact energy production and metabolic flux .
Molecular Mechanism
The molecular mechanism of action of this compound involves its ability to interact with biomolecules through redox reactions. This compound can bind to enzymes and proteins, either inhibiting or activating their function depending on the context . For example, it can inhibit the activity of certain oxidases by competing with their natural substrates. Additionally, this compound can induce changes in gene expression by modulating transcription factors and other regulatory proteins .
Temporal Effects in Laboratory Settings
In laboratory settings, the effects of this compound change over time due to its stability and degradation properties. This compound is relatively stable under standard laboratory conditions, but it can degrade over time, especially in the presence of light and oxygen . Long-term exposure to this compound can lead to sustained oxidative stress in cells, which may result in chronic changes in cellular function and metabolism .
Dosage Effects in Animal Models
The effects of this compound vary with different dosages in animal models. At low doses, this compound can enhance cellular function by promoting redox balance and activating stress response pathways . At high doses, it can induce toxic effects, such as oxidative damage to cellular components and disruption of metabolic processes . Threshold effects have been observed, where the beneficial effects of this compound are only seen within a specific dosage range.
Metabolic Pathways
This compound is involved in several metabolic pathways, including those related to oxidative stress and redox regulation. It interacts with enzymes such as superoxide dismutase and catalase, which are involved in the detoxification of reactive oxygen species . This compound can also affect metabolic flux by altering the levels of key metabolites, such as NADH and NADPH, which are essential for cellular energy production and redox balance .
Transport and Distribution
Within cells and tissues, this compound is transported and distributed through interactions with transporters and binding proteins. It can be taken up by cells via passive diffusion or active transport mechanisms . Once inside the cell, this compound can localize to specific compartments, such as the mitochondria and nucleus, where it exerts its effects on cellular function and metabolism .
Subcellular Localization
The subcellular localization of this compound is critical for its activity and function. This compound can be directed to specific organelles, such as the mitochondria, through targeting signals and post-translational modifications . In the mitochondria, this compound can influence mitochondrial function and energy production by modulating the redox state and interacting with mitochondrial enzymes . Its localization to the nucleus can also impact gene expression by affecting transcription factors and other regulatory proteins.
Preparation Methods
Synthetic Routes and Reaction Conditions
Keto-ABNO can be synthesized through the aerobic oxidation of 9-azabicyclo[3.3.1]nonane. The process involves using a catalyst system that includes copper (I) and Keto-ABNO itself. This method is highly effective for the oxidation of secondary alcohols, including unactivated aliphatic substrates .
Industrial Production Methods
In industrial settings, Keto-ABNO is produced using similar catalytic systems but on a larger scale. The process involves the use of acidic solvents and co-catalysts to achieve high yields. For example, the aerobic oxidation of 5-hydroxymethylfurfural to 2,5-diformylfuran using Keto-ABNO as a catalyst in acetic acid has been reported to achieve a 93.4% yield within half an hour at room temperature .
Chemical Reactions Analysis
Types of Reactions
Keto-ABNO primarily undergoes oxidation reactions. It is highly effective in the aerobic oxidation of alcohols to aldehydes or ketones and the oxidation of secondary amines to imines .
Common Reagents and Conditions
The common reagents used in these reactions include copper (I) salts and bismuth nitrate. The reactions are typically carried out at room temperature and ambient pressure, making them convenient and environmentally friendly .
Major Products Formed
The major products formed from these reactions include aldehydes, ketones, and imines. For instance, the oxidation of secondary alcohols using Keto-ABNO results in the formation of the corresponding ketones .
Scientific Research Applications
Keto-ABNO has a wide range of applications in scientific research:
Chemistry: It is used as a catalyst in various oxidation reactions, including the oxidation of alcohols and amines.
Medicine: It is employed in the synthesis of pharmaceuticals and antifungal agents.
Industry: Keto-ABNO is used in the production of high-value chemicals from biomass, such as 2,5-diformylfuran.
Comparison with Similar Compounds
Similar Compounds
TEMPO (2,2,6,6-Tetramethylpiperidine 1-oxyl): Another widely used oxidation catalyst, but Keto-ABNO is more efficient due to less steric hindrance.
ABNO (9-azabicyclo[3.3.1]nonane N-oxyl): Similar to Keto-ABNO but lacks the ketone functional group, making Keto-ABNO more versatile in certain reactions.
Uniqueness
Keto-ABNO is unique due to its high efficiency in oxidation reactions and its stability under various conditions. It is more effective than TEMPO in oxidizing secondary amines to imines and alcohols to ketones or carboxylic acids .
Properties
InChI |
InChI=1S/C8H12NO2/c10-8-4-6-2-1-3-7(5-8)9(6)11/h6-7H,1-5H2 | |
---|---|---|
Source | PubChem | |
URL | https://pubchem.ncbi.nlm.nih.gov | |
Description | Data deposited in or computed by PubChem | |
InChI Key |
AMZBXNVXJGUYMF-UHFFFAOYSA-N | |
Source | PubChem | |
URL | https://pubchem.ncbi.nlm.nih.gov | |
Description | Data deposited in or computed by PubChem | |
Canonical SMILES |
C1CC2CC(=O)CC(C1)N2[O] | |
Source | PubChem | |
URL | https://pubchem.ncbi.nlm.nih.gov | |
Description | Data deposited in or computed by PubChem | |
Molecular Formula |
C8H12NO2 | |
Source | PubChem | |
URL | https://pubchem.ncbi.nlm.nih.gov | |
Description | Data deposited in or computed by PubChem | |
DSSTOX Substance ID |
DTXSID30991538 | |
Record name | (3-Oxo-9-azabicyclo[3.3.1]nonan-9-yl)oxidanyl | |
Source | EPA DSSTox | |
URL | https://comptox.epa.gov/dashboard/DTXSID30991538 | |
Description | DSSTox provides a high quality public chemistry resource for supporting improved predictive toxicology. | |
Molecular Weight |
154.19 g/mol | |
Source | PubChem | |
URL | https://pubchem.ncbi.nlm.nih.gov | |
Description | Data deposited in or computed by PubChem | |
CAS No. |
7123-92-4 | |
Record name | Norpseudopelleterine-N-oxyl | |
Source | ChemIDplus | |
URL | https://pubchem.ncbi.nlm.nih.gov/substance/?source=chemidplus&sourceid=0007123924 | |
Description | ChemIDplus is a free, web search system that provides access to the structure and nomenclature authority files used for the identification of chemical substances cited in National Library of Medicine (NLM) databases, including the TOXNET system. | |
Record name | (3-Oxo-9-azabicyclo[3.3.1]nonan-9-yl)oxidanyl | |
Source | EPA DSSTox | |
URL | https://comptox.epa.gov/dashboard/DTXSID30991538 | |
Description | DSSTox provides a high quality public chemistry resource for supporting improved predictive toxicology. | |
Retrosynthesis Analysis
AI-Powered Synthesis Planning: Our tool employs the Template_relevance Pistachio, Template_relevance Bkms_metabolic, Template_relevance Pistachio_ringbreaker, Template_relevance Reaxys, Template_relevance Reaxys_biocatalysis model, leveraging a vast database of chemical reactions to predict feasible synthetic routes.
One-Step Synthesis Focus: Specifically designed for one-step synthesis, it provides concise and direct routes for your target compounds, streamlining the synthesis process.
Accurate Predictions: Utilizing the extensive PISTACHIO, BKMS_METABOLIC, PISTACHIO_RINGBREAKER, REAXYS, REAXYS_BIOCATALYSIS database, our tool offers high-accuracy predictions, reflecting the latest in chemical research and data.
Strategy Settings
Precursor scoring | Relevance Heuristic |
---|---|
Min. plausibility | 0.01 |
Model | Template_relevance |
Template Set | Pistachio/Bkms_metabolic/Pistachio_ringbreaker/Reaxys/Reaxys_biocatalysis |
Top-N result to add to graph | 6 |
Feasible Synthetic Routes
Q1: What is 9-azabicyclo[3.3.1]nonan-3-one N-oxyl (ketoABNO) and what makes it useful in organic synthesis?
A1: this compound is an organic nitroxyl radical that acts as a highly effective catalyst for the aerobic oxidation of alcohols to aldehydes or ketones. [, , ] This compound exhibits remarkable catalytic activity when combined with copper(I) salts, facilitating the oxidation of various alcohols, including challenging unactivated aliphatic substrates. [] The reaction utilizes molecular oxygen as the terminal oxidant, leading to the generation of water as the sole byproduct, which highlights its environmentally benign nature. []
Q2: Can you elaborate on the reaction mechanism of this compound-catalyzed alcohol oxidations?
A2: While the exact mechanism is still under investigation, studies suggest that this compound, in conjunction with a copper(I) catalyst, undergoes a redox cycle to facilitate alcohol oxidation. It is proposed that the copper(I) species activates molecular oxygen, generating a reactive copper-oxygen species. This species then interacts with this compound, forming a this compound+ species capable of oxidizing the alcohol substrate. The resulting aldehyde or ketone product is released, and the this compound catalyst is regenerated, enabling catalytic turnover. []
Q3: What are the advantages of employing a this compound/NOx cocatalytic system for aldehyde oxidation?
A3: The use of this compound in combination with a NOx source like sodium nitrite provides a mild and efficient method for the aerobic oxidation of aldehydes to carboxylic acids. [] This methodology exhibits excellent functional group tolerance and is compatible with a wide range of substrates, including those bearing sensitive functionalities. The mild reaction conditions minimize racemization, enabling the synthesis of valuable chiral carboxylic acids, especially when combined with asymmetric hydroformylation reactions. []
Disclaimer and Information on In-Vitro Research Products
Please be aware that all articles and product information presented on BenchChem are intended solely for informational purposes. The products available for purchase on BenchChem are specifically designed for in-vitro studies, which are conducted outside of living organisms. In-vitro studies, derived from the Latin term "in glass," involve experiments performed in controlled laboratory settings using cells or tissues. It is important to note that these products are not categorized as medicines or drugs, and they have not received approval from the FDA for the prevention, treatment, or cure of any medical condition, ailment, or disease. We must emphasize that any form of bodily introduction of these products into humans or animals is strictly prohibited by law. It is essential to adhere to these guidelines to ensure compliance with legal and ethical standards in research and experimentation.