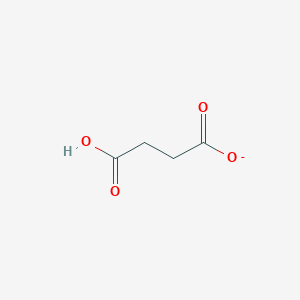
Hydrogen succinate
Overview
Description
Hydrogen succinate, or monosodium succinate, is the monoester or monosalt of succinic acid (HOOC-CH₂-CH₂-COOH), where one carboxylic acid group is neutralized (e.g., as a sodium salt) or esterified, leaving the other group free. It is widely utilized in pharmaceuticals, agrochemicals, and polymer chemistry due to its dual functionality (acid/ester) and biocompatibility. For example, ribociclib this compound crystallizes in a layered structure (space group P-1) with alternating cationic and anionic layers . Its hydrogen-bonding capacity enables interactions with biological targets, such as succinate dehydrogenase (SDH), and enhances solubility in drug formulations .
Scientific Research Applications
Pharmaceutical Applications
Drug Formulations
Hydrogen succinate is frequently used as a salt form in pharmaceuticals to enhance solubility and stability. For instance, ribociclib this compound has been studied for its crystal structure and interactions, revealing insights into its stability and efficacy as an anticancer agent. The crystal structure analysis indicates that the hydrogen bonds within the compound significantly influence its physical properties and biological activity .
Case Study: Ribociclib this compound
- Structure : The compound crystallizes in a specific space group, with defined intermolecular hydrogen bonds that stabilize the structure.
- Findings : The study confirmed the importance of hydrogen bonding in maintaining the integrity of the drug formulation, which is crucial for its therapeutic effectiveness .
Material Science
Nonlinear Optical Applications
Rubidium this compound has been developed into single crystals for nonlinear optical applications. These crystals exhibit favorable optical properties, including high transparency and a notable energy band gap, making them suitable for photonic devices .
Property | Value |
---|---|
Transparency | 89% |
Cut-off Wavelength | 240 nm |
Energy Band Gap | 5.21 eV |
Case Study: Rubidium this compound Hydrate
- Growth Technique : Crystals were grown using the slow evaporation solution growth technique.
- Applications : The resulting crystals are being explored for use in advanced photonic devices, particularly in solar-blind ultraviolet applications .
Biochemical Research
Metabolic Studies
this compound serves as an important intermediate in various metabolic pathways. Its role in energy production and cellular respiration makes it a focus of research in biochemistry.
Case Study: Biohydrogenation Processes
Research has shown that this compound is produced during the biohydrogenation of fatty acids by rumen fungi. This process highlights its significance in ruminant nutrition and microbial metabolism .
Analytical Chemistry
High-Performance Liquid Chromatography (HPLC)
this compound derivatives are often analyzed using HPLC due to their relevance in pharmaceutical quality control. For example, methylprednisolone this compound is routinely assessed for potency and purity using HPLC methodologies.
Application Area | Description |
---|---|
Pharmaceutical Analysis | Quality control of drug substances |
Clinical Diagnosis | Estimation of metabolites in body fluids |
Q & A
Basic Research Questions
Q. What experimental methodologies are recommended for synthesizing and characterizing hydrogen succinate derivatives in the laboratory?
- Methodological Answer : Synthesis should follow protocols validated for succinate esters or salts, emphasizing purity control via techniques like HPLC (High-Performance Liquid Chromatography) or NMR (Nuclear Magnetic Resonance). For characterization, use spectroscopic methods (FTIR, UV-Vis) to confirm functional groups and crystallography (e.g., SHELX programs for structure refinement) to resolve molecular geometry . Ensure reproducibility by documenting solvent systems, stoichiometric ratios, and reaction kinetics. Purity verification should align with pharmacopeial standards if applicable .
Q. How can researchers determine the solubility and stability of this compound in aqueous vs. non-polar solvents?
- Methodological Answer : Conduct phase-solubility studies using gravimetric or spectrophotometric methods under controlled pH and temperature. Stability assays should include accelerated degradation studies (e.g., 40°C/75% RH) with HPLC monitoring. Compare results against thermodynamic models (e.g., van’t Hoff analysis) to predict behavior in biological or environmental systems .
Q. What statistical approaches are appropriate for analyzing the impact of synthesis parameters (e.g., concentration, pressure) on this compound product yield?
- Methodological Answer : Employ factorial design (e.g., 3-factor full factorial) to test variables like reactant concentration, temperature, and agitation rate. Use ANOVA (Analysis of Variance) to identify significant factors and optimize conditions. For example, a study on polybutylene succinate (PBS) used this method to determine optimal air pressure (4 bar) and feed rate (80 mL/h) for nanofiber production .
Advanced Research Questions
Q. How can contradictions in this compound’s role in mitochondrial function (e.g., pro- vs. anti-oxidant effects) be resolved through experimental design?
- Methodological Answer : Design dose-response studies using isolated mitochondria to measure hydrogen peroxide (H₂O₂) production at varying succinate concentrations. Employ phylogenetic independent contrasts (PIC) to account for species-specific metabolic variations, as seen in lifespan studies correlating H₂O₂ levels with maximum longevity . Validate findings with redox-sensitive fluorescent probes (e.g., MitoSOX) and cross-reference with transcriptomic data on succinate dehydrogenase (SDH) activity .
Q. What strategies are effective for analyzing hydrogen bonding patterns in this compound crystals to predict supramolecular assembly?
- Methodological Answer : Apply graph set analysis (GSA) to categorize hydrogen bond motifs (e.g., chains, rings) in crystallographic data. Use SHELXL for refinement and Mercury software for visualization. For instance, Etter’s framework identifies donor-acceptor distances and angles, critical for designing co-crystals with enhanced bioavailability .
Q. How can researchers optimize this compound-based polymers (e.g., PBS) for biomedical applications while ensuring biodegradability?
- Methodological Answer : Combine solution blow spinning (SBS) with green chemistry principles to fabricate nanofibers. Characterize mechanical properties via tensile testing and biodegradation rates using soil burial assays. A study achieved minimal fiber diameter (156 nm) by optimizing PBS concentration (9% w/w) and air pressure, ensuring eco-friendly scalability .
Q. Methodological Challenges and Data Analysis
Q. What are the best practices for reconciling discrepancies between computational models and experimental data in this compound interactions?
- Methodological Answer : Perform molecular dynamics (MD) simulations with force fields parameterized for carboxylate groups (e.g., AMBER). Validate against experimental data (e.g., DSC for thermal behavior, XRD for crystallinity). Use sensitivity analysis to identify model limitations, such as neglecting solvent effects or non-covalent interactions .
Q. How should researchers structure literature reviews to address gaps in this compound’s pharmacological mechanisms?
- Methodological Answer : Follow PICOT criteria (Population, Intervention, Comparison, Outcome, Time) to frame hypotheses. For example: “In in vitro models (P), does this compound (I) compared to fumarate (C) alter SDH activity (O) within 24 hours (T)?” Systematically screen databases (PubMed, SciFinder) and prioritize primary studies over reviews .
Comparison with Similar Compounds
Succinic Acid and Diesters
- Succinic Acid : The parent dicarboxylic acid (HOOC-CH₂-CH₂-COOH) serves as a metabolic intermediate in the tricarboxylic acid cycle. Unlike hydrogen succinate, both carboxylic groups are free, limiting its use in hydrophobic formulations.
- Diethyl Succinate : A diester (CH₂CH₂-OOC-CH₂-CH₂-COO-CH₂CH₃) formed by esterifying both carboxylic groups. It is prominent in wine aroma profiles, with concentrations influenced by barrel storage (e.g., 157.8 µg/L in EMLT barrels for Merlot wine) . Diethyl succinate lacks hydrogen-bonding capacity compared to this compound, reducing its interaction with enzymes like SDH.
Poly(alkylene succinate)s
These biodegradable polyesters, synthesized from succinic acid and diols (e.g., ethylene glycol, 1,4-butanediol), exhibit distinct thermal and mechanical properties:
Polyester | Tm (°C) | Tg (°C) | Decomposition Temp (°C) |
---|---|---|---|
Poly(ethylene succinate) | 64.2 | -20.1 | 420–430 |
Poly(butylene succinate) | 117.8 | -45.3 | 420–430 |
Poly(decylene succinate) | 85.6 | -60.2 | 420–430 |
All decompose via β-hydrogen bond scission, but this compound’s smaller size allows faster enzymatic hydrolysis, making it more suitable for drug delivery than high-molecular-weight polyesters .
Succinate Dehydrogenase Inhibitors (SDHIs)
This compound is a natural substrate of SDH, binding via hydrogen bonds to residues like Trp173 and Tyr56. Synthetic SDHIs mimic this interaction:
Compound | Target Fungus | EC₅₀ (µg/mL) | Key Interactions with SDH |
---|---|---|---|
This compound | B. cinerea | N/A | Native substrate binding |
Compound 3f | B. cinerea | 0.046 | Hydrogen bonds with Ser17, Ser39 |
Compound 7d | R. solani | 0.046 | Hydrogen bonds with Trp173, Tyr58 |
Thifluzamide | B. cinerea | 0.741 | Hydrophobic interactions |
Pyrazole carboxamides (e.g., compound 3f) and benzoylurea derivatives (e.g., compound 4l) achieve higher antifungal activity than natural succinate by forming additional hydrogen bonds and hydrophobic interactions .
Prodrugs and Drug Delivery Systems
- Succinate Prodrugs : NV189 (bis-(1-acetoxy-ethyl) succinate) is a cell-permeable prodrug that releases succinate intracellularly, bypassing mitochondrial complex I defects. Its ester groups enhance lipophilicity compared to this compound .
- TPGS (Tocopherol PEG Succinate): Used in nanomedicine, TPGS links α-tocopherol to PEG via a succinate group. The succinate linker stabilizes hydrogen bonds with P-glycoprotein (e.g., Lys515, Ser1077), improving drug bioavailability by 2–3 fold compared to non-succinate PEG .
Solubility in Organic Solvents
This compound derivatives like metoprolol succinate exhibit solubility in alcohols (ethanol, propanol) due to hydrogen bonding. DFT studies show bond distances between metoprolol succinate and methanol (2.156 Å), correlating with higher solubility compared to sterically hindered isopropanol (2.256 Å) .
Preparation Methods
Acid-Catalyzed Esterification with Hydrochloride Salts
Reaction Mechanism and Process Design
Industrial-scale production of succinate esters often employs acid-catalyzed esterification. As detailed in a patent by CN109053442A ( ), succinic acid reacts with alcohols (e.g., methanol, ethanol, or butanol) in the presence of hydrochloride salts such as magnesium chloride (MgCl₂), calcium chloride (CaCl₂), or zinc chloride (ZnCl₂). The reaction proceeds via nucleophilic acyl substitution, where the alcohol attacks the protonated carbonyl group of succinic acid, forming an ester and water. The hydrochloride salt acts as a Lewis acid catalyst, polarizing the carbonyl group and enhancing reaction kinetics.
Key reaction conditions include:
-
Temperature : 20–150°C (optimized at 50–100°C for energy efficiency)
-
Pressure : 1–5 bar (ambient pressure preferred for cost-effectiveness)
-
Catalyst concentration : ≥5 wt% of the reaction mixture to ensure phase separation and high yield ( ).
Role of Hydrochloride Salts in Phase Separation
The inclusion of MgCl₂ or ZnCl₂ significantly improves product isolation. These salts increase the ionic strength of the aqueous phase, reducing the solubility of non-polar succinate esters and facilitating liquid-liquid separation. For example, dibutyl succinate forms a distinct organic layer in the presence of 10 wt% MgCl₂, enabling >90% recovery without distillation ( ).
Recycling and Sustainability
The process integrates a closed-loop system for hydrochloride recovery. Post-reaction, the aqueous phase containing dissolved MgCl₂ is thermally decomposed at 350–600°C via pyrohydrolysis, regenerating magnesia (MgO) and hydrogen chloride (HCl). The HCl is recycled into the esterification reactor, while MgO is reused in fermentation processes to neutralize succinic acid, minimizing waste ( ).
Catalytic Hydrogenation of Maleic Acid Esters
Hydrogenation Process Overview
An alternative route to succinate esters involves the hydrogenation of maleic acid diesters. As disclosed in CN102001939A ( ), maleic acid diesters (e.g., dimethyl maleate) are subjected to hydrogen gas in a fixed-bed reactor packed with a hydrogenation catalyst (e.g., palladium on carbon or Raney nickel). The exothermic reaction saturates the double bond in maleate, yielding succinate diesters with >99.6% purity:
3O2C-CH=CH-CO2CH3 + \text{H}2 \rightarrow \text{CH}3O2C-CH2-CH2-CO2CH_3 \quad
Catalyst Optimization and Product Recycling
Catalyst longevity is enhanced by recycling a portion of the product stream. For instance, 20–30% of the output succinate diester is mixed with fresh maleate feedstock, diluting impurities and maintaining consistent reactor performance. This approach reduces catalyst deactivation and extends operational cycles to over 1,000 hours ( ).
Economic and Environmental Considerations
Hydrogenation offers advantages in raw material costs, as maleic anhydride—a precursor to maleate esters—is cheaper than bio-based succinic acid. However, the method requires high-purity hydrogen and precise temperature control to avoid side reactions, such as over-hydrogenation to butanediol derivatives ( ).
Synthesis of Monoalkyl Succinates
Selective Esterification Techniques
Monoalkyl succinates, such as mono-methyl succinate, are synthesized through controlled esterification. A patent (CN104557536A, ) outlines a method using stoichiometric imbalances to favor monoester formation. By limiting the alcohol-to-succinic-acid ratio to 1:1 and employing mild catalysts (e.g., p-toluenesulfonic acid), monoesters are obtained with 70–80% selectivity. Excess alcohol or higher temperatures shift equilibrium toward diester formation, necessitating precise process control.
Industrial Applications
Monoesters serve as intermediates in surfactants and biodegradable plastics. For example, mono-methyl succinate is esterified further with long-chain alcohols to produce emulsifiers for agrochemicals ( ).
Pharmaceutical Derivative Synthesis
Hemisuccinate Ester Formation
Hydrogen succinate derivatives, such as hydrocortisone this compound (HHS), are critical in drug formulation. HHS is synthesized by reacting hydrocortisone with succinic anhydride in a base-catalyzed esterification:
The reaction typically achieves 85–90% yield under anhydrous conditions, with dimethylaminopyridine (DMAP) accelerating acylation.
Purification and Characterization
Crude HHS is purified via recrystallization from ethanol-water mixtures, yielding pharmaceutical-grade material with ≤0.1% residual solvents. Quality control includes HPLC analysis to verify <2% diastereomeric impurities ( ).
Comparative Analysis of Preparation Methods
Method | Yield | Catalyst | Energy Demand | Scalability |
---|---|---|---|---|
Acid-catalyzed esterification | 85–95% | MgCl₂, ZnCl₂ | Moderate | High (batch/continuous) |
Catalytic hydrogenation | >99% | Pd/C, Raney Ni | High | Moderate |
Monoester synthesis | 70–80% | p-TsOH | Low | Lab-scale |
Key Findings :
-
Acid-catalyzed esterification is preferred for bulk production due to its closed-loop recycling and low catalyst costs ( ).
-
Hydrogenation excels in purity but faces challenges in hydrogen storage and catalyst expenses ( ).
-
Pharmaceutical derivatives require stringent purification, limiting throughput but ensuring compliance with regulatory standards ( ).
Properties
Molecular Formula |
C4H5O4- |
---|---|
Molecular Weight |
117.08 g/mol |
IUPAC Name |
4-hydroxy-4-oxobutanoate |
InChI |
InChI=1S/C4H6O4/c5-3(6)1-2-4(7)8/h1-2H2,(H,5,6)(H,7,8)/p-1 |
InChI Key |
KDYFGRWQOYBRFD-UHFFFAOYSA-M |
SMILES |
C(CC(=O)[O-])C(=O)O |
Canonical SMILES |
C(CC(=O)[O-])C(=O)O |
Origin of Product |
United States |
Retrosynthesis Analysis
AI-Powered Synthesis Planning: Our tool employs the Template_relevance Pistachio, Template_relevance Bkms_metabolic, Template_relevance Pistachio_ringbreaker, Template_relevance Reaxys, Template_relevance Reaxys_biocatalysis model, leveraging a vast database of chemical reactions to predict feasible synthetic routes.
One-Step Synthesis Focus: Specifically designed for one-step synthesis, it provides concise and direct routes for your target compounds, streamlining the synthesis process.
Accurate Predictions: Utilizing the extensive PISTACHIO, BKMS_METABOLIC, PISTACHIO_RINGBREAKER, REAXYS, REAXYS_BIOCATALYSIS database, our tool offers high-accuracy predictions, reflecting the latest in chemical research and data.
Strategy Settings
Precursor scoring | Relevance Heuristic |
---|---|
Min. plausibility | 0.01 |
Model | Template_relevance |
Template Set | Pistachio/Bkms_metabolic/Pistachio_ringbreaker/Reaxys/Reaxys_biocatalysis |
Top-N result to add to graph | 6 |
Feasible Synthetic Routes
Disclaimer and Information on In-Vitro Research Products
Please be aware that all articles and product information presented on BenchChem are intended solely for informational purposes. The products available for purchase on BenchChem are specifically designed for in-vitro studies, which are conducted outside of living organisms. In-vitro studies, derived from the Latin term "in glass," involve experiments performed in controlled laboratory settings using cells or tissues. It is important to note that these products are not categorized as medicines or drugs, and they have not received approval from the FDA for the prevention, treatment, or cure of any medical condition, ailment, or disease. We must emphasize that any form of bodily introduction of these products into humans or animals is strictly prohibited by law. It is essential to adhere to these guidelines to ensure compliance with legal and ethical standards in research and experimentation.