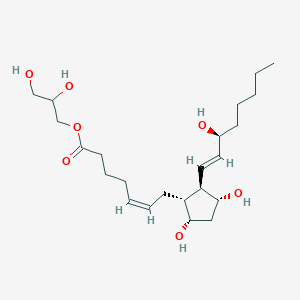
prostaglandin F2alpha 1-glyceryl ester
Overview
Description
Prostaglandin F2.alpha.-1-glyceryl ester is a naturally occurring prostaglandin derivative. Prostaglandins are a group of physiologically active lipid compounds having diverse hormone-like effects in animals. Prostaglandin F2.alpha.-1-glyceryl ester is specifically known for its role in various biological processes, including inflammation and smooth muscle function .
Mechanism of Action
Target of Action
PGF2alpha-G primarily targets the Prostaglandin F2alpha receptor (FP) . This receptor is a G protein-coupled receptor (GPCR) that is required for female reproductive function such as luteolysis and parturition . It has also been implicated in blood pressure regulation, atherosclerosis, and other inflammation-related disorders .
Mode of Action
PGF2alpha-G acts by binding to the FP receptor . It is released in response to an increase in oxytocin levels in the uterus, and stimulates both luteolytic activity and the release of oxytocin . This interaction with its targets results in changes in cellular processes such as cell proliferation, differentiation, and apoptosis .
Biochemical Pathways
The biochemical pathways affected by PGF2alpha-G involve the synthesis of prostanoids, including prostaglandin (PG) E2, PGD2, prostacyclin (PGI2), thromboxane A2 (TxA2), and PGF2alpha . These are generated through PGH synthase (PGHS), commonly known as cyclooxygenase (COX), in response to a wide variety of stimuli acting in a paracrine or autocrine manner . The biological functions of PGs could be modulated at multiple levels such as COX, PG synthases, and downstream receptors .
Pharmacokinetics
The elimination half-life of PGF2alpha is less than 1 minute in blood plasma . This suggests that PGF2alpha-G may also have a short half-life and rapid clearance, which could impact its bioavailability.
Result of Action
Through the FP receptor, PGF2alpha-G promotes the expression of c-fos, atrial natriuretic factor (ANF), and alpha-skeletal actin in cardiomyocytes . It induces cardiac myocyte hypertrophy in vitro and cardiac growth in rats
Action Environment
The action of PGF2alpha-G can be influenced by environmental factors. For instance, reactive oxygen species (ROS) play important roles in regulating the luteolytic action of PGF2alpha . The local concentration of ROS is controlled by superoxide dismutase (SOD), the main enzyme involved in the control of intraluteal ROS . Thus, the action, efficacy, and stability of PGF2alpha-G can be influenced by the balance of ROS and SOD in the environment.
Biochemical Analysis
Biochemical Properties
Prostaglandin F2alpha 1-glyceryl ester interacts with various enzymes, proteins, and other biomolecules in biochemical reactions . For instance, it is involved in the chemoenzymatic total synthesis of prostaglandins, guided by biocatalytic retrosynthesis . An unprecedented Baeyer–Villiger monooxygenase (BVMO)-catalyzed stereoselective oxidation and a ketoreductase (KRED)-catalyzed diastereoselective reduction of enones are utilized in combination to set the critical stereochemical configurations under mild conditions .
Cellular Effects
The effects of this compound on various types of cells and cellular processes are significant. It influences cell function, including impacts on cell signaling pathways, gene expression, and cellular metabolism . For example, it acts on its specific and distinct cell surface G protein-coupled receptors (GPCRs) or peroxisome proliferator-activated receptors (PPARs) .
Molecular Mechanism
This compound exerts its effects at the molecular level through various mechanisms. It binds with biomolecules, inhibits or activates enzymes, and changes gene expression . For instance, it is involved in the stereoselective synthesis of prostaglandins, setting critical stereochemical configurations under mild conditions .
Metabolic Pathways
This compound is involved in various metabolic pathways. It interacts with enzymes or cofactors, and may affect metabolic flux or metabolite levels . For example, it is involved in the chemoenzymatic total synthesis of prostaglandins .
Preparation Methods
Synthetic Routes and Reaction Conditions: Prostaglandin F2.alpha.-1-glyceryl ester can be synthesized by incubating 2-arachidonoyl glycerol with cyclooxygenase-2 and specific prostaglandin H2 isomerases in cell cultures . This process involves the conversion of 2-arachidonoyl glycerol to prostaglandin H2, which is then isomerized to form prostaglandin F2.alpha.-1-glyceryl ester.
Industrial Production Methods: The industrial production of prostaglandin F2.alpha.-1-glyceryl ester typically involves large-scale cell culture systems where the enzymatic conversion of 2-arachidonoyl glycerol to prostaglandin F2.alpha.-1-glyceryl ester is optimized for yield and purity .
Chemical Reactions Analysis
Types of Reactions: Prostaglandin F2.alpha.-1-glyceryl ester undergoes several types of chemical reactions, including oxidation and isomerization .
Common Reagents and Conditions:
Oxidation: Prostaglandin F2.alpha.-1-glyceryl ester can be oxidized by 15-hydroxyprostaglandin dehydrogenase.
Isomerization: The 2-glyceryl ester moiety can equilibrate rapidly with the more stable 1-glyceryl ester in typical aqueous media.
Major Products: The major products formed from these reactions include various prostaglandin derivatives and metabolites, such as prostaglandin H2 and other glyceryl esters .
Scientific Research Applications
Prostaglandin F2.alpha.-1-glyceryl ester has several scientific research applications:
Comparison with Similar Compounds
- Prostaglandin E2-1-glyceryl ester
- Prostaglandin D2-1-glyceryl ester
- Thromboxane A-2-glyceryl ester
Comparison: Prostaglandin F2.alpha.-1-glyceryl ester is unique in its specific biological functions and receptor interactions compared to other prostaglandin glyceryl esters. While prostaglandin E2-1-glyceryl ester and prostaglandin D2-1-glyceryl ester have distinct roles in inflammation and immune responses, prostaglandin F2.alpha.-1-glyceryl ester is more involved in smooth muscle function and related physiological processes .
Properties
IUPAC Name |
2,3-dihydroxypropyl 7-[3,5-dihydroxy-2-(3-hydroxyoct-1-enyl)cyclopentyl]hept-5-enoate | |
---|---|---|
Details | Computed by LexiChem 2.6.6 (PubChem release 2019.06.18) | |
Source | PubChem | |
URL | https://pubchem.ncbi.nlm.nih.gov | |
Description | Data deposited in or computed by PubChem | |
InChI |
InChI=1S/C23H40O7/c1-2-3-6-9-17(25)12-13-20-19(21(27)14-22(20)28)10-7-4-5-8-11-23(29)30-16-18(26)15-24/h4,7,12-13,17-22,24-28H,2-3,5-6,8-11,14-16H2,1H3 | |
Details | Computed by InChI 1.0.5 (PubChem release 2019.06.18) | |
Source | PubChem | |
URL | https://pubchem.ncbi.nlm.nih.gov | |
Description | Data deposited in or computed by PubChem | |
InChI Key |
NWKPOVHSHWJQNI-UHFFFAOYSA-N | |
Details | Computed by InChI 1.0.5 (PubChem release 2019.06.18) | |
Source | PubChem | |
URL | https://pubchem.ncbi.nlm.nih.gov | |
Description | Data deposited in or computed by PubChem | |
Canonical SMILES |
CCCCCC(C=CC1C(CC(C1CC=CCCCC(=O)OCC(CO)O)O)O)O | |
Details | Computed by OEChem 2.1.5 (PubChem release 2019.06.18) | |
Source | PubChem | |
URL | https://pubchem.ncbi.nlm.nih.gov | |
Description | Data deposited in or computed by PubChem | |
Molecular Formula |
C23H40O7 | |
Details | Computed by PubChem 2.1 (PubChem release 2019.06.18) | |
Source | PubChem | |
URL | https://pubchem.ncbi.nlm.nih.gov | |
Description | Data deposited in or computed by PubChem | |
DSSTOX Substance ID |
DTXSID20693998 | |
Record name | 2,3-Dihydroxypropyl 9,11,15-trihydroxyprosta-5,13-dien-1-oate | |
Source | EPA DSSTox | |
URL | https://comptox.epa.gov/dashboard/DTXSID20693998 | |
Description | DSSTox provides a high quality public chemistry resource for supporting improved predictive toxicology. | |
Molecular Weight |
428.6 g/mol | |
Details | Computed by PubChem 2.1 (PubChem release 2021.05.07) | |
Source | PubChem | |
URL | https://pubchem.ncbi.nlm.nih.gov | |
Description | Data deposited in or computed by PubChem | |
CAS No. |
43042-79-1 | |
Record name | 2,3-Dihydroxypropyl 9,11,15-trihydroxyprosta-5,13-dien-1-oate | |
Source | EPA DSSTox | |
URL | https://comptox.epa.gov/dashboard/DTXSID20693998 | |
Description | DSSTox provides a high quality public chemistry resource for supporting improved predictive toxicology. | |
Retrosynthesis Analysis
AI-Powered Synthesis Planning: Our tool employs the Template_relevance Pistachio, Template_relevance Bkms_metabolic, Template_relevance Pistachio_ringbreaker, Template_relevance Reaxys, Template_relevance Reaxys_biocatalysis model, leveraging a vast database of chemical reactions to predict feasible synthetic routes.
One-Step Synthesis Focus: Specifically designed for one-step synthesis, it provides concise and direct routes for your target compounds, streamlining the synthesis process.
Accurate Predictions: Utilizing the extensive PISTACHIO, BKMS_METABOLIC, PISTACHIO_RINGBREAKER, REAXYS, REAXYS_BIOCATALYSIS database, our tool offers high-accuracy predictions, reflecting the latest in chemical research and data.
Strategy Settings
Precursor scoring | Relevance Heuristic |
---|---|
Min. plausibility | 0.01 |
Model | Template_relevance |
Template Set | Pistachio/Bkms_metabolic/Pistachio_ringbreaker/Reaxys/Reaxys_biocatalysis |
Top-N result to add to graph | 6 |
Feasible Synthetic Routes
Disclaimer and Information on In-Vitro Research Products
Please be aware that all articles and product information presented on BenchChem are intended solely for informational purposes. The products available for purchase on BenchChem are specifically designed for in-vitro studies, which are conducted outside of living organisms. In-vitro studies, derived from the Latin term "in glass," involve experiments performed in controlled laboratory settings using cells or tissues. It is important to note that these products are not categorized as medicines or drugs, and they have not received approval from the FDA for the prevention, treatment, or cure of any medical condition, ailment, or disease. We must emphasize that any form of bodily introduction of these products into humans or animals is strictly prohibited by law. It is essential to adhere to these guidelines to ensure compliance with legal and ethical standards in research and experimentation.