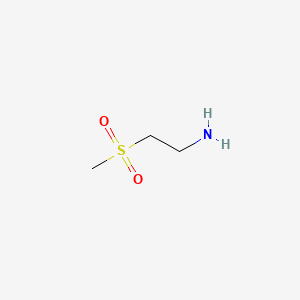
2-Aminoethylmethyl sulfone
Overview
Description
2-Aminoethylmethyl sulfone (CAS 49773-20-8) is a sulfone-containing organic compound with the molecular formula C₃H₉NO₂S and a molecular weight of 123.176 g/mol. Structurally, it consists of an ethylamine backbone substituted with a methylsulfonyl group (-SO₂CH₃) at the second carbon, giving it the IUPAC name 2-(methylsulfonyl)ethanamine . Key physical properties include a density of 1.223 g/cm³, boiling point of 368.5°C, and a polar surface area (PSA) of 68.54 Ų, which contributes to its solubility in polar solvents .
Preparation Methods
Traditional Synthetic Routes and Their Limitations
Diborane-Mediated Reduction
Early synthetic approaches for 2-aminoethylmethyl sulfone relied on diborane (B₂H₆) as a chemoselective reducing agent. This method involved the reduction of 2-methylsulfonylethyl nitrile intermediates to yield the target compound. However, this route faced critical limitations:
-
High Cost : Diborane’s expense and handling complexity made large-scale production economically unfeasible .
-
Purification Challenges : The hydrochloride salt form of this compound produced via this method exhibited poor crystallinity, necessitating laborious purification steps .
-
Environmental Concerns : Diborane’s toxicity and the generation of hazardous byproducts raised significant safety and disposal issues .
Novel Catalytic Hydrogenation Method
Reaction Pathway and Intermediate Synthesis
A groundbreaking method described in EP2305634A1 bypasses diborane by employing catalytic hydrogenation (Figure 1) . The process involves two key stages:
Stage 1: Synthesis of 2-(N,N-Dibenzylamino)ethyl Methyl Sulfone
-
Starting Material : 2’-(Methyl sulfone) ethyl methylbenzenesulfonate.
-
Substitution Reaction : Reacted with N,N-dibenzylamine in ethanol at room temperature to form 2-(N,N-dibenzylamino)ethyl methyl sulfone .
-
Advantages : Ambient conditions eliminate the need for specialized equipment, while ethanol serves as a green solvent .
Stage 2: Catalytic Hydrogenation
-
Catalysts : Raney nickel (Ni), palladium on carbon (Pd/C), or platinum dioxide (PtO₂).
-
Conditions : Hydrogen gas at 1–3 atm pressure in alcoholic solvents (e.g., methanol, ethanol) .
-
Outcome : Selective removal of benzyl groups yields this compound with >85% purity .
Table 1: Optimized Conditions for Catalytic Hydrogenation
Parameter | Optimal Value | Impact on Yield/Purity |
---|---|---|
Catalyst | Pd/C (10 wt%) | 89% yield, minimal byproducts |
Hydrogen Pressure | 2 atm | Balances reaction rate/safety |
Solvent | Methanol | Enhances intermediate solubility |
Temperature | 25–30°C | Prevents thermal degradation |
Advantages Over Traditional Methods
-
Cost Efficiency : Eliminates diborane, reducing reagent costs by ~40% .
-
Scalability : Adaptable to industrial-scale reactors with consistent batch yields .
-
Environmental Impact : Ethanol and methanol solvents are recyclable, aligning with green chemistry principles .
Salt Formation and Purification Techniques
Hydrochloride Salt Synthesis
The hydrochloride salt of this compound is pivotal for pharmaceutical formulations due to its enhanced stability. The novel method achieves this via:
-
Acid-Base Reaction : Freebase this compound is treated with hydrochloric acid (HCl) in ethanol, yielding the hydrochloride salt .
-
Crystallization : Slow cooling induces high-purity crystals (99.5% purity), avoiding traditional recrystallization pitfalls .
Alternative Salt Derivatives
Recent patents describe synthesizing carboxylate and phosphate salts to modulate solubility and bioavailability:
-
Carboxylate Salts : Reacting the freebase with carboxylic acids (e.g., acetic acid) under reflux .
-
Phosphate Salts : Utilizing phosphoric acid in aqueous ethanol, enabling tailored dissolution profiles .
Table 2: Comparative Properties of this compound Salts
Salt Type | Solubility (mg/mL) | Stability (25°C) | Application Context |
---|---|---|---|
Hydrochloride | 12.3 | >24 months | Solid dosage formulations |
Acetate | 45.6 | 18 months | Injectable solutions |
Phosphate | 32.1 | 12 months | Buffered oral suspensions |
Industrial-Scale Production Protocols
Process Optimization for Large Batches
Industrial adoption of the catalytic hydrogenation method requires modifications for efficiency:
-
Continuous Flow Reactors : Reduce reaction time by 30% compared to batch systems .
-
Catalyst Recycling : Pd/C catalysts are recovered via filtration, achieving 95% reuse efficiency .
-
In-Line Purification : Integrated crystallization units directly yield pharmaceutical-grade product, bypassing intermediate isolation .
Environmental and Economic Metrics
Chemical Reactions Analysis
Types of Reactions
2-Aminoethylmethyl sulfone undergoes various types of chemical reactions, including:
Oxidation: The sulfone group can be further oxidized to form sulfoxides or other higher oxidation states.
Reduction: The compound can be reduced to form sulfides or thiols under specific conditions.
Substitution: The amino group can participate in nucleophilic substitution reactions, leading to the formation of various derivatives.
Common Reagents and Conditions
Oxidation: Common oxidizing agents include hydrogen peroxide and potassium permanganate.
Reduction: Reducing agents such as lithium aluminum hydride or sodium borohydride are often used.
Substitution: Reagents like alkyl halides or acyl chlorides are used in substitution reactions.
Major Products Formed
The major products formed from these reactions depend on the specific conditions and reagents used. For example, oxidation can yield sulfoxides, while reduction can produce sulfides. Substitution reactions can lead to a variety of aminoethyl derivatives .
Scientific Research Applications
Pharmaceutical Applications
1.1 Drug Synthesis Intermediates
2-Aminoethylmethyl sulfone serves as a crucial intermediate in the synthesis of various pharmaceuticals. Notably, it has been identified in the synthesis of lapatinib, a tyrosine kinase inhibitor used in breast cancer treatment. The amino sulfone side chain of lapatinib enhances its bioactivity by providing essential hydrogen-bonding sites that facilitate binding to target enzymes .
1.2 Anti-Cancer Compounds
Research indicates that this compound is integral to the development of anti-cancer agents. Its derivatives are being explored for their potential to inhibit tumor growth and metastasis. For instance, the compound is involved in synthesizing other sulfonamide-based drugs that exhibit anti-tumor properties .
Preparation Methods
The preparation of this compound and its salts can be achieved through various synthetic routes. These methods not only enhance yield but also aim to reduce environmental impact:
2.1 Novel Synthetic Routes
Recent patents describe innovative methods for synthesizing this compound using environmentally friendly processes. One method involves reacting sulfonates with ammonia or amines at room temperature, which facilitates the formation of the desired compound while minimizing waste .
2.2 Salt Formation Techniques
The formation of different salts from this compound is crucial for improving solubility and stability. Techniques include transforming the hydrochloride salt into free alkali and reacting it with various acids to yield salts such as carboxylates and phosphates . This versatility allows for tailored applications in pharmaceutical formulations.
Case Studies and Research Findings
3.1 Case Study: Lapatinib Synthesis
In a study assessing sustainable manufacturing routes for lapatinib, researchers demonstrated that integrating this compound into the synthetic pathway significantly improved efficiency and reduced solvent usage. The study highlighted the compound's role in forming stable imine intermediates during synthesis, which can be further reduced to yield the final product with high purity .
3.2 Comparative Analysis of Synthesis Methods
A comparative analysis of traditional versus novel synthesis methods for this compound revealed that newer approaches not only lower costs but also enhance overall yield and environmental sustainability. The integration of catalytic hydrogenation techniques has shown promise in improving reaction conditions and product stability .
Data Summary Table
Application Area | Description | Key Findings |
---|---|---|
Drug Synthesis | Intermediate for anti-cancer drugs | Vital for lapatinib synthesis |
Anti-Cancer Research | Potential inhibitors of tumor growth | Derivatives show promising bioactivity |
Preparation Methods | Environmentally friendly synthesis routes | Novel methods improve yield and reduce waste |
Salt Formation | Enhances solubility and stability | Versatile applications in pharmaceutical formulations |
Mechanism of Action
The mechanism of action of 2-Aminoethylmethyl sulfone involves its interaction with specific molecular targets, such as enzymes or receptors. The amino group can form hydrogen bonds with active sites of enzymes, while the sulfone group can participate in various chemical interactions, enhancing the compound’s binding affinity and specificity .
Comparison with Similar Compounds
Structural Analogues: Sulfonamides and Thiazole Derivatives
2-Aminoethylmethyl sulfone shares structural motifs with sulfonamides and thiazole-based compounds, which are known for their biological activities. For instance:
- N-butyl-benzenesulfonamide and famphur (organophosphate insecticide) exhibit structural similarity scores of 0.309 and 0.203, respectively, to the metabolite E2, which may share functional groups with this compound .
- Thiabendazole (antifungal) and 5-benzyl-1,3-thiazol-2-amine (thiazole derivative) show similarity scores of 0.791 and 0.876 to metabolite AB2, highlighting the relevance of sulfone and amine groups in bioactivity .
Functional Group Comparison: Sulfone-Containing Drugs
- Sulindac Sulfone: A non-steroidal anti-inflammatory drug (NSAID) metabolite, sulindac sulfone inhibits mTOR signaling by binding to voltage-dependent anion channels (VDAC1/2). The sulfone group is critical for VDAC interaction, a trait shared with this compound .
- WEHI-9625 : This antiapoptotic compound also contains a sulfone moiety, suggesting that sulfones may broadly enhance binding to ion channels or enzymes .
Research Gap: While sulindac sulfone’s VDAC binding is well-documented, this compound’s effects on ion channels remain unstudied .
Physicochemical Properties vs. Other Sulfones
Notable Trends:
- Solubility : All three compounds exhibit high solubility in polar solvents due to sulfone and amine groups.
- Molecular Weight: this compound’s lower molecular weight may enhance membrane permeability compared to bulkier analogues like CAS 10027-70-0 .
Biological Activity
2-Aminoethylmethyl sulfone (AEMS), also known as methylsulfonyl-2-aminoethanol, is a sulfone compound with significant biological activity. Its structural characteristics allow it to interact with various biological molecules, influencing cellular processes and metabolic pathways. This article provides a comprehensive overview of the biological activity of AEMS, including its mechanisms of action, effects on cellular metabolism, and potential therapeutic applications.
Chemical Structure and Properties
The molecular formula of AEMS is C3H9NO2S, and it features a sulfone group that contributes to its reactivity and biological interactions. The compound's functional groups enable it to participate in various biochemical reactions, particularly those involving enzyme modulation.
AEMS acts primarily as a competitive antagonist in biochemical pathways, particularly in the synthesis of folic acid. It mimics p-aminobenzoic acid (PABA), which is essential for bacterial DNA synthesis. By inhibiting this pathway, AEMS can effectively suppress bacterial growth.
Key Mechanisms:
- Inhibition of Folic Acid Synthesis : AEMS interferes with the enzymatic processes that convert PABA into folic acid, crucial for nucleotide synthesis in bacteria.
- Stimulation of ATP-Dependent Enzymes : Studies indicate that AEMS enhances the activity of ATP-dependent enzymes, leading to increased ATP production, which is vital for cellular energy metabolism.
Biological Activity and Effects
AEMS exhibits a range of biological activities that impact cellular functions:
- Cellular Metabolism : AEMS has been shown to enhance ATP production in various cell types, thereby improving overall cellular metabolism. This effect is dose-dependent; lower doses promote metabolic activity while higher doses may lead to cytotoxic effects.
- Gene Expression Modulation : The compound influences gene expression related to energy metabolism and stress responses. It has been observed to alter the expression levels of genes involved in mitochondrial function.
Table 1: Summary of Biological Activities of AEMS
Activity Type | Description | Reference |
---|---|---|
Inhibition of Bacterial Growth | Acts as a PABA analog, inhibiting folic acid synthesis | |
ATP Production Enhancement | Stimulates ATP-dependent enzymes | |
Gene Expression Modulation | Alters expression related to metabolism |
Case Studies and Research Findings
Several studies have investigated the biological effects of AEMS:
- Antibacterial Activity : Research has demonstrated that AEMS shows significant antibacterial properties against both Gram-positive and Gram-negative bacteria. In vitro studies indicated that it was more effective against Staphylococcus aureus compared to Escherichia coli .
- Toxicological Studies : Animal models have shown that while lower doses of AEMS enhance metabolic functions, higher doses can lead to adverse effects such as cellular damage and disruption of metabolic pathways.
- Therapeutic Applications : Due to its ability to inhibit bacterial growth and modulate metabolic pathways, AEMS is being explored for potential therapeutic applications in treating bacterial infections and metabolic disorders .
Transport and Distribution
The transport mechanisms for AEMS involve specific transport proteins that facilitate its entry into cells. Once inside, it localizes primarily in the cytoplasm and mitochondria, where it exerts its effects on energy metabolism and gene expression.
Q & A
Basic Research Questions
Q. What are the established synthetic routes for 2-aminoethylmethyl sulfone, and how do reaction conditions influence yield and purity?
- The synthesis of sulfone derivatives like this compound often involves oxidation of thioethers or nucleophilic substitution reactions. A green strategy using electrochemical methods (e.g., cyclic voltammetry in aqueous buffers) has been reported for sulfone synthesis, minimizing hazardous reagents . Key factors include pH, temperature, and catalyst selection. For example, acidic conditions (pH 2.0) enhance reaction efficiency in electrochemical synthesis . Purification typically employs recrystallization or column chromatography, with purity verified via HPLC or melting point analysis .
Q. How can researchers confirm the structural integrity of this compound derivatives?
- Single-crystal X-ray diffraction is the gold standard for structural confirmation, providing atomic-level resolution of bond lengths and angles (e.g., mean σ(C–C) = 0.003 Å) . Complementary techniques include:
- NMR spectroscopy : To verify sulfone (-SO₂-) and amine (-NH₂) functional groups.
- Mass spectrometry : For molecular weight validation (e.g., C₃H₁₀ClNO₂S has a molecular weight of 159.635 g/mol) .
- Elemental analysis : To confirm empirical formulas .
Q. What are the key physicochemical properties of this compound relevant to experimental design?
- Critical properties include:
- Melting point : 170°C (pure compound) .
- Solubility : High solubility in polar solvents (e.g., water, DMSO) due to the sulfone group’s polarity.
- Stability : Hydrolytically stable under neutral conditions but may degrade in strong acids/bases .
Advanced Research Questions
Q. How does the sulfone moiety in this compound influence its biological activity, particularly in enzyme inhibition studies?
- The sulfone group enhances electrophilicity, enabling interactions with nucleophilic residues in enzyme active sites. For example, sulfone-containing compounds like (Methanesulfonyl-phenethyl-amino)-acetic acid act as enzyme inhibitors by mimicking transition states or blocking substrate binding . Researchers should perform kinetic assays (e.g., IC₅₀ determination) and molecular docking simulations to map binding interactions.
Q. What strategies resolve contradictions in data when studying sulfone derivatives’ electrochemical behavior?
- Contradictions in cyclic voltammetry (CV) data (e.g., oxidation peaks) may arise from impurities or solvent effects. Mitigation strategies include:
- Reproducibility checks : Replicate experiments under controlled conditions (temperature, electrolyte concentration) .
- Statistical analysis : Use tools like ANOVA to assess significance of observed variations .
- Cross-validation : Compare CV results with computational models (e.g., density functional theory) .
Q. How can researchers optimize the synthesis of this compound for scalability in academic labs?
- Scalability requires balancing yield, safety, and cost. Recommendations:
- Flow chemistry : Continuous synthesis reduces reaction time and improves consistency .
- Catalyst optimization : Use immobilized catalysts for easy recovery and reuse.
- In-line monitoring : Implement techniques like FTIR to track reaction progress in real time .
Q. What are the best practices for analyzing sulfone derivatives in complex mixtures (e.g., biological matrices)?
- Advanced chromatographic methods are essential:
- HPLC-MS/MS : Provides high sensitivity and specificity for quantifying sulfones in biological samples .
- Derivatization : Enhance detection by converting amines to fluorescent or UV-active derivatives (e.g., dansyl chloride tagging) .
Q. Methodological Notes
- Data Reporting : Include raw data tables in appendices and processed data (e.g., crystallographic R factors, statistical outputs) in the main text to ensure transparency .
- Safety Protocols : Handle this compound hydrochloride (CAS 104458-24-4) with PPE due to potential irritancy; refer to MSDS for storage guidelines (e.g., dry, inert atmosphere) .
Properties
IUPAC Name |
2-methylsulfonylethanamine | |
---|---|---|
Source | PubChem | |
URL | https://pubchem.ncbi.nlm.nih.gov | |
Description | Data deposited in or computed by PubChem | |
InChI |
InChI=1S/C3H9NO2S/c1-7(5,6)3-2-4/h2-4H2,1H3 | |
Source | PubChem | |
URL | https://pubchem.ncbi.nlm.nih.gov | |
Description | Data deposited in or computed by PubChem | |
InChI Key |
SDNXQWUJWNTDCC-UHFFFAOYSA-N | |
Source | PubChem | |
URL | https://pubchem.ncbi.nlm.nih.gov | |
Description | Data deposited in or computed by PubChem | |
Canonical SMILES |
CS(=O)(=O)CCN | |
Source | PubChem | |
URL | https://pubchem.ncbi.nlm.nih.gov | |
Description | Data deposited in or computed by PubChem | |
Molecular Formula |
C3H9NO2S | |
Source | PubChem | |
URL | https://pubchem.ncbi.nlm.nih.gov | |
Description | Data deposited in or computed by PubChem | |
DSSTOX Substance ID |
DTXSID80198035 | |
Record name | 2-Aminoethylmethyl sulfone | |
Source | EPA DSSTox | |
URL | https://comptox.epa.gov/dashboard/DTXSID80198035 | |
Description | DSSTox provides a high quality public chemistry resource for supporting improved predictive toxicology. | |
Molecular Weight |
123.18 g/mol | |
Source | PubChem | |
URL | https://pubchem.ncbi.nlm.nih.gov | |
Description | Data deposited in or computed by PubChem | |
CAS No. |
49773-20-8 | |
Record name | 2-(Methylsulfonyl)ethanamine | |
Source | CAS Common Chemistry | |
URL | https://commonchemistry.cas.org/detail?cas_rn=49773-20-8 | |
Description | CAS Common Chemistry is an open community resource for accessing chemical information. Nearly 500,000 chemical substances from CAS REGISTRY cover areas of community interest, including common and frequently regulated chemicals, and those relevant to high school and undergraduate chemistry classes. This chemical information, curated by our expert scientists, is provided in alignment with our mission as a division of the American Chemical Society. | |
Explanation | The data from CAS Common Chemistry is provided under a CC-BY-NC 4.0 license, unless otherwise stated. | |
Record name | 2-Aminoethylmethyl sulfone | |
Source | ChemIDplus | |
URL | https://pubchem.ncbi.nlm.nih.gov/substance/?source=chemidplus&sourceid=0049773208 | |
Description | ChemIDplus is a free, web search system that provides access to the structure and nomenclature authority files used for the identification of chemical substances cited in National Library of Medicine (NLM) databases, including the TOXNET system. | |
Record name | 2-Aminoethylmethyl sulfone | |
Source | EPA DSSTox | |
URL | https://comptox.epa.gov/dashboard/DTXSID80198035 | |
Description | DSSTox provides a high quality public chemistry resource for supporting improved predictive toxicology. | |
Retrosynthesis Analysis
AI-Powered Synthesis Planning: Our tool employs the Template_relevance Pistachio, Template_relevance Bkms_metabolic, Template_relevance Pistachio_ringbreaker, Template_relevance Reaxys, Template_relevance Reaxys_biocatalysis model, leveraging a vast database of chemical reactions to predict feasible synthetic routes.
One-Step Synthesis Focus: Specifically designed for one-step synthesis, it provides concise and direct routes for your target compounds, streamlining the synthesis process.
Accurate Predictions: Utilizing the extensive PISTACHIO, BKMS_METABOLIC, PISTACHIO_RINGBREAKER, REAXYS, REAXYS_BIOCATALYSIS database, our tool offers high-accuracy predictions, reflecting the latest in chemical research and data.
Strategy Settings
Precursor scoring | Relevance Heuristic |
---|---|
Min. plausibility | 0.01 |
Model | Template_relevance |
Template Set | Pistachio/Bkms_metabolic/Pistachio_ringbreaker/Reaxys/Reaxys_biocatalysis |
Top-N result to add to graph | 6 |
Feasible Synthetic Routes
Disclaimer and Information on In-Vitro Research Products
Please be aware that all articles and product information presented on BenchChem are intended solely for informational purposes. The products available for purchase on BenchChem are specifically designed for in-vitro studies, which are conducted outside of living organisms. In-vitro studies, derived from the Latin term "in glass," involve experiments performed in controlled laboratory settings using cells or tissues. It is important to note that these products are not categorized as medicines or drugs, and they have not received approval from the FDA for the prevention, treatment, or cure of any medical condition, ailment, or disease. We must emphasize that any form of bodily introduction of these products into humans or animals is strictly prohibited by law. It is essential to adhere to these guidelines to ensure compliance with legal and ethical standards in research and experimentation.