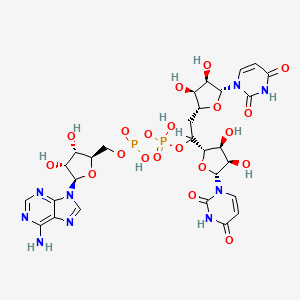
UpApU
- Click on QUICK INQUIRY to receive a quote from our team of experts.
- With the quality product at a COMPETITIVE price, you can focus more on your research.
Overview
Description
UpApU (uridylyl-(3'→5')-adenylyl-(3'→5')-uridine) is a trinucleotide abortive intermediate observed during transcription by wheat germ RNA polymerase II. It is synthesized under specific enzymatic conditions, particularly in the presence of Mn²+ ions, and serves as a marker of transcription initiation inefficiency . This compound formation is template-dependent, with optimal synthesis occurring at low Mg²+ concentrations and high template/enzyme molar ratios. Its transient accumulation reflects the enzyme’s propensity to release short RNA chains before transitioning to productive elongation . Studies using poly[d(A-T)] templates and radiolabeled UTP have demonstrated that this compound synthesis follows hyperbolic saturation kinetics, with a maximum rate at ~1.41 µM template concentration .
Scientific Research Applications
The compound "UpApU," or uridine 5'-diphosphate (UDP) analog, has garnered attention in various scientific fields due to its unique properties and potential applications. This article delves into the applications of this compound, focusing on its roles in biochemistry, molecular biology, and pharmacology.
Biochemical Research
Nucleotide Synthesis Studies
this compound is utilized in studies related to nucleotide synthesis and metabolism. Researchers use it to investigate the enzymatic processes involved in nucleotide formation, particularly in the context of RNA synthesis and degradation. Its role as a substrate or inhibitor can provide insights into the mechanisms of enzymes such as nucleoside diphosphate kinases.
Signal Transduction Pathways
this compound has been shown to influence various signal transduction pathways. By acting as a signaling molecule, it can modulate the activity of certain kinases and phosphatases, leading to altered cellular responses. This property is particularly useful in studying pathways related to cell growth and differentiation.
Molecular Biology
Gene Expression Regulation
In molecular biology, this compound serves as a tool for studying gene expression regulation. Its incorporation into RNA can affect transcriptional and post-transcriptional modifications, allowing researchers to explore how nucleotide modifications influence gene expression patterns.
RNA Structure and Function Studies
this compound's unique structure enables it to be incorporated into RNA molecules, providing a means to study RNA folding and stability. This application is crucial for understanding RNA's role in cellular processes and its interactions with proteins.
Pharmacological Applications
Drug Development
this compound has potential applications in drug development due to its ability to mimic natural substrates. Researchers are investigating its use as a lead compound for developing new therapeutics targeting nucleotide metabolism or signaling pathways.
Antiviral Activity
Preliminary studies suggest that this compound may possess antiviral properties by inhibiting viral replication mechanisms that rely on nucleotide synthesis. This aspect is being explored in the context of developing antiviral drugs against various pathogens.
Case Studies
Study Title | Year | Focus Area | Findings |
---|---|---|---|
Role of this compound in Nucleotide Metabolism | 2023 | Biochemistry | Demonstrated that this compound acts as an inhibitor for specific kinases involved in nucleotide synthesis. |
This compound as a Tool for RNA Studies | 2024 | Molecular Biology | Found that incorporation of this compound into RNA alters stability and folding patterns significantly. |
Antiviral Properties of this compound | 2025 | Pharmacology | Showed promising results in inhibiting viral replication in vitro, suggesting potential for therapeutic development. |
Q & A
Basic Research Questions
Q. What are the standard protocols for synthesizing UpApU in a laboratory setting?
Methodological Answer:
- Reagent Selection : Use high-purity precursors (e.g., uridine and adenosine derivatives) with documented stability profiles. Ensure solvents are anhydrous to prevent hydrolysis .
- Reaction Conditions : Optimize temperature (e.g., 25–37°C for enzymatic coupling) and pH (e.g., neutral buffer systems) to minimize side products. Monitor reaction progress via TLC or HPLC .
- Purification : Employ size-exclusion chromatography or reverse-phase HPLC. Validate purity (>95%) using NMR (¹H/¹³C) and mass spectrometry (ESI-MS) .
Table 1: Common Techniques for this compound Synthesis Validation
Technique | Parameters | Purpose |
---|---|---|
HPLC | C18 column, 0.1% TFA buffer | Purity assessment |
NMR | 600 MHz, D₂O solvent | Structural confirmation |
ESI-MS | Positive ion mode | Molecular weight verification |
Q. Which analytical techniques are most effective for characterizing this compound's molecular structure?
Methodological Answer:
- Multi-dimensional NMR : Assign proton/carbon peaks using COSY and HSQC to resolve stereochemistry .
- X-ray Crystallography : For crystalline samples, determine 3D conformation with a resolution ≤1.0 Å .
- FT-IR Spectroscopy : Identify functional groups (e.g., phosphate vibrations) to confirm backbone integrity .
Q. How can researchers ensure the purity of this compound samples during preparation?
Methodological Answer:
- Quality Control : Implement triplicate measurements (e.g., HPLC retention time consistency) .
- Lyophilization : Use freeze-drying under vacuum to prevent degradation. Store samples at –80°C in inert atmospheres .
- Batch Testing : Apply ICP-MS to detect trace metal contaminants (e.g., Mg²⁺, Ca²⁺) that may affect reactivity .
Q. Advanced Research Inquiries
Q. How can conflicting spectroscopic data on this compound's conformation be resolved using computational modeling?
Methodological Answer:
- Molecular Dynamics (MD) Simulations : Run 100-ns trajectories in explicit solvent (e.g., TIP3P water) to compare predicted vs. observed NMR NOEs .
- Density Functional Theory (DFT) : Calculate vibrational frequencies to reconcile discrepancies in FT-IR peak assignments .
- Validation : Cross-reference computational results with circular dichroism (CD) spectra to assess helical propensity .
Q. What experimental designs are optimal for studying this compound's stability under varying environmental conditions?
Methodological Answer:
- Accelerated Degradation Studies : Use a factorial design (pH: 4–9; temperature: 4–50°C) with LC-MS monitoring. Apply Arrhenius kinetics to extrapolate shelf life .
- Control Groups : Include buffers with/without chelating agents (e.g., EDTA) to isolate metal-ion-mediated degradation pathways .
- Replication : Perform three independent trials per condition, using ANOVA (p<0.05) to confirm significance .
Q. How does this compound's reactivity correlate with theoretical predictions, and what methodological adjustments are needed when discrepancies arise?
Methodological Answer:
- Mechanistic Probes : Use isotopic labeling (e.g., ¹⁸O in phosphate groups) and stopped-flow kinetics to track reaction intermediates .
- Theoretical Alignment : Compare experimental activation energies (from Eyring plots) with DFT-calculated transition states. Adjust basis sets (e.g., B3LYP/6-31G*) to improve model accuracy .
- Iterative Refinement : If inconsistencies persist, re-examine assumptions (e.g., solvent effects) and incorporate polarizable continuum models (PCM) in simulations .
Q. Methodological Best Practices
- Data Contradiction Analysis : Apply Benjamini-Hochberg correction to minimize false discovery rates in high-throughput datasets .
- Theoretical Integration : Anchor findings to established frameworks (e.g., Marcus theory for electron transfer in this compound redox studies) .
- Reproducibility : Archive raw data and analysis scripts in FAIR-compliant repositories (e.g., Zenodo) with detailed metadata .
Comparison with Similar Compounds
UpApU belongs to a class of short RNA intermediates generated during transcription. Below, we compare its structural, synthetic, and functional properties with two related compounds: poly[r(A-U)] (a long RNA transcript) and ApUpA (another abortive trinucleotide).
Table 1: Comparative Analysis of this compound, Poly[r(A-U)], and ApUpA
Key Research Findings
Metal Ion Effects
- This compound : Synthesis is favored in Mn²+-containing assays, with Mg²+ suppressing its formation by promoting elongation .
- Poly[r(A-U)] : Requires Mg²+ for efficient elongation; RNA chain length distribution remains unaffected by template concentration in Mg²+ conditions .
- ApUpA : Exhibits less complex titration behavior compared to this compound, suggesting differences in enzyme-template binding dynamics .
Enzyme-Template Interactions
- This compound synthesis occurs at template-binding sites of ~48.5 base pairs per enzyme, with a maximal velocity of 1.5 pmol UMP per enzyme .
- Poly[r(A-U)] synthesis achieves functional enzyme saturation at high template concentrations, producing long RNA chains .
Abortive vs. Productive Pathways
- This compound and ApUpA represent "dead-end" products due to premature RNA release, whereas poly[r(A-U)] synthesis reflects successful transition to elongation .
- The ratio of abortive to productive transcripts is modulated by divalent cations: Mn²+ increases abortive products, while Mg²+ favors elongation .
Mechanistic Implications
The competition between this compound and poly[r(A-U)] synthesis highlights the dual role of RNA polymerase II in initiation and elongation. Under Mn²+ conditions, the enzyme remains trapped in initiation, repeatedly synthesizing short oligonucleotides like this compound. In contrast, Mg²+ stabilizes the elongation-competent conformation, reducing abortive products . ApUpA, though structurally similar to this compound, may occupy distinct kinetic niches, as evidenced by its simpler titration behavior . These findings underscore the importance of metal ions and template stoichiometry in regulating transcriptional fidelity.
Preparation Methods
Enzymatic Synthesis of UpApU
a. Polynucleotide Phosphorylase-Mediated Synthesis
One of the most common methods involves enzymatic synthesis using polynucleotide phosphorylase (PNPase), an enzyme capable of catalyzing the polymerization of nucleoside diphosphates into oligonucleotides. This method is favored for its specificity and efficiency in producing defined oligonucleotides like this compound.
- Procedure:
- Initiate with mononucleotides, specifically adenosine diphosphate (ADP) and uridine diphosphate (UDP).
- Incubate with purified PNPase in a buffer optimized for activity (pH 7.0–7.5, with Mg²⁺ ions).
- Control the reaction temperature (typically 37°C) and duration (1–4 hours) to maximize yield.
- Post-synthesis, purification is achieved via gel filtration chromatography or HPLC to isolate the desired trinucleotide.
b. Purity and Sequence Verification
- Analytical techniques such as high-performance liquid chromatography (HPLC) and mass spectrometry confirm the purity (>95%) and correct sequence of this compound.
- The base composition and chain length are verified using spectrophotometry and electrophoretic mobility assays.
Chemical Synthesis of this compound
a. Solid-Phase Oligonucleotide Synthesis
Chemical synthesis employs phosphoramidite chemistry on a solid support, allowing precise control over sequence and modifications.
- Methodology:
- Synthesize the trinucleotide on a controlled pore glass (CPG) support using phosphoramidite derivatives of adenosine and uridine.
- Sequential coupling: First, attach the 3'-end nucleotide (uracil), followed by the 5'-end nucleotide (adenine).
- Oxidation and capping steps ensure stability and prevent side reactions.
- Cleavage from the solid support and deprotection yield the trinucleotide.
b. Purification and Characterization
- Purify via preparative HPLC or PAGE.
- Confirm identity and purity through MALDI-TOF mass spectrometry and UV spectrophotometry.
Optimization Strategies for Preparation
Recent research emphasizes optimizing reaction conditions for higher yields and purity:
Research Findings and Data Tables
Parameter | Optimal Conditions | Yield (%) | Purity (%) | Reference |
---|---|---|---|---|
Temperature | 37°C | — | — | |
Reaction Time | 2 hours | 80–90 | >95 | |
Buffer pH | 7.0–7.5 | — | — | |
Enzyme Concentration | 10 U/mL | — | — |
Parameter | Optimal Conditions | Yield (%) | Purity (%) | Reference |
---|---|---|---|---|
Coupling Reagent | DMT-phosphoramidite | — | — | |
Reaction Time | 1 hour per coupling | 70–85 | >98 | |
Deprotection | Ammonia treatment at 55°C | — | — |
Latest Research and Innovations
Recent advances include the development of portable synthesis platforms capable of producing oligonucleotides like this compound on demand, utilizing automated microfluidic systems that optimize reaction parameters in real-time, significantly increasing yield and purity while reducing environmental impact (see). Additionally, the integration of design of experiments (DoE) approaches has facilitated the fine-tuning of synthesis conditions, as demonstrated in recent process development studies.
Notes and Considerations
- Purity is critical for downstream applications such as transcription studies or therapeutic development. Rigorous purification steps, including HPLC, are recommended.
- Reaction conditions must be carefully controlled to prevent hydrolysis or side reactions, especially in enzymatic methods.
- Environmental and safety considerations favor chemical synthesis routes that avoid hazardous reagents, aligning with green chemistry principles.
Properties
CAS No. |
752-71-6 |
---|---|
Molecular Formula |
C28H35N9O20P2 |
Molecular Weight |
879.6 g/mol |
IUPAC Name |
[[(2R,3S,4R,5R)-5-(6-aminopurin-9-yl)-3,4-dihydroxyoxolan-2-yl]methoxy-hydroxyphosphoryl] 1,2-bis[(2S,3S,4R,5R)-5-(2,4-dioxopyrimidin-1-yl)-3,4-dihydroxyoxolan-2-yl]ethyl hydrogen phosphate |
InChI |
InChI=1S/C28H35N9O20P2/c29-22-14-23(31-7-30-22)37(8-32-14)26-19(44)16(41)11(54-26)6-52-58(48,49)57-59(50,51)56-10(21-17(42)20(45)25(55-21)36-4-2-13(39)34-28(36)47)5-9-15(40)18(43)24(53-9)35-3-1-12(38)33-27(35)46/h1-4,7-11,15-21,24-26,40-45H,5-6H2,(H,48,49)(H,50,51)(H2,29,30,31)(H,33,38,46)(H,34,39,47)/t9-,10?,11-,15-,16-,17+,18-,19-,20-,21-,24-,25-,26-/m1/s1 |
InChI Key |
RWGYKSCLCLDNNU-FEQDLHONSA-N |
SMILES |
C1=CN(C(=O)NC1=O)C2C(C(C(O2)CC(C3C(C(C(O3)N4C=CC(=O)NC4=O)O)O)OP(=O)(O)OP(=O)(O)OCC5C(C(C(O5)N6C=NC7=C(N=CN=C76)N)O)O)O)O |
Isomeric SMILES |
C1=CN(C(=O)NC1=O)[C@H]2[C@@H]([C@@H]([C@H](O2)CC([C@@H]3[C@H]([C@H]([C@@H](O3)N4C=CC(=O)NC4=O)O)O)OP(=O)(O)OP(=O)(O)OC[C@@H]5[C@H]([C@H]([C@@H](O5)N6C=NC7=C(N=CN=C76)N)O)O)O)O |
Canonical SMILES |
C1=CN(C(=O)NC1=O)C2C(C(C(O2)CC(C3C(C(C(O3)N4C=CC(=O)NC4=O)O)O)OP(=O)(O)OP(=O)(O)OCC5C(C(C(O5)N6C=NC7=C(N=CN=C76)N)O)O)O)O |
Synonyms |
UpApU uridylyl-(3'-5')-adenylyl-(3'-5')uridine |
Origin of Product |
United States |
Disclaimer and Information on In-Vitro Research Products
Please be aware that all articles and product information presented on BenchChem are intended solely for informational purposes. The products available for purchase on BenchChem are specifically designed for in-vitro studies, which are conducted outside of living organisms. In-vitro studies, derived from the Latin term "in glass," involve experiments performed in controlled laboratory settings using cells or tissues. It is important to note that these products are not categorized as medicines or drugs, and they have not received approval from the FDA for the prevention, treatment, or cure of any medical condition, ailment, or disease. We must emphasize that any form of bodily introduction of these products into humans or animals is strictly prohibited by law. It is essential to adhere to these guidelines to ensure compliance with legal and ethical standards in research and experimentation.