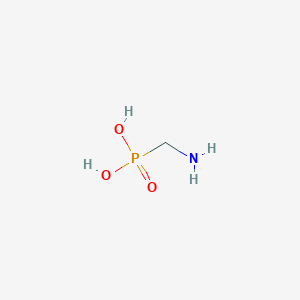
(Aminomethyl)phosphonic acid
Overview
Description
The compound “AMEP” refers to a dihydropyridine calcium antagonist that inhibits the transmembrane influx of calcium ions into cardiac and vascular smooth muscle. It has a greater affinity towards vascular smooth muscle than cardiac muscle, making it a peripheral vasodilator that reduces peripheral vascular resistance and lowers blood pressure .
Mechanism of Action
Target of Action
(Aminomethyl)phosphonic acid (AMPA) is a weak organic acid with a phosphonic acid group . It is primarily used as a biocide and pesticide . AMPA is also used in research to assess the exposure of glyphosate , a widely used herbicide. The primary targets of AMPA are therefore organisms that are harmful to crops and other plants.
Mode of Action
AMPA is one of the primary degradation products of the herbicide glyphosate . It interacts with its targets by mimicking the phosphates and carboxylates of biological molecules, potentially inhibiting metabolic enzymes . This interaction results in the disruption of essential biological processes in the target organisms, leading to their death.
Biochemical Pathways
AMPA affects the biochemical pathways of its target organisms by inhibiting key metabolic enzymes . It is also a degradation product of glyphosate, and it can be broken down further by manganese oxide under laboratory conditions . In soil, manganese oxide is usually only present in trace amounts . Microbial degradation of AMPA is the more likely degradation pathway, where it degrades into phosphoric acid and ultimately to carbon dioxide and inorganic phosphate .
Result of Action
The result of AMPA’s action is the death of its target organisms. By inhibiting key metabolic enzymes, AMPA disrupts essential biological processes, leading to the death of harmful organisms . AMPA has toxicity which is comparable to that of glyphosate and it is therefore considered to be of similar toxicological concern .
Action Environment
The action of AMPA is influenced by environmental factors. For example, the presence of manganese oxide in the environment can affect the degradation of AMPA . Additionally, the microbial composition of the soil can influence the degradation pathway of AMPA . The efficacy and stability of AMPA may also be affected by factors such as temperature, pH, and the presence of other chemicals in the environment.
Preparation Methods
Synthetic Routes and Reaction Conditions
The synthesis of AMEP involves the preparation of dihydropyridine derivatives. The reaction typically includes the condensation of an aldehyde with a β-keto ester in the presence of ammonia or an amine. The reaction conditions often involve refluxing the mixture in an organic solvent such as ethanol or methanol.
Industrial Production Methods
Industrial production of AMEP follows similar synthetic routes but on a larger scale. The process is optimized for high yield and purity, often involving continuous flow reactors and automated systems to control reaction parameters precisely.
Chemical Reactions Analysis
Types of Reactions
AMEP undergoes several types of chemical reactions, including:
Oxidation: AMEP can be oxidized to form corresponding pyridine derivatives.
Reduction: Reduction of AMEP can lead to the formation of tetrahydropyridine derivatives.
Substitution: AMEP can undergo substitution reactions where functional groups on the dihydropyridine ring are replaced by other groups.
Common Reagents and Conditions
Oxidation: Common oxidizing agents include potassium permanganate and chromium trioxide.
Reduction: Reducing agents such as lithium aluminum hydride or sodium borohydride are used.
Substitution: Reagents like halogens or alkylating agents are used under conditions that favor nucleophilic substitution.
Major Products
Oxidation: Pyridine derivatives.
Reduction: Tetrahydropyridine derivatives.
Substitution: Various substituted dihydropyridine derivatives.
Scientific Research Applications
AMEP has a wide range of applications in scientific research:
Chemistry: Used as a model compound to study the behavior of calcium channel blockers.
Biology: Investigated for its effects on cellular calcium dynamics.
Medicine: Used in the treatment of hypertension and angina. It is also studied for its potential in treating other cardiovascular diseases.
Industry: Utilized in the development of new pharmaceuticals and as a reference compound in quality control.
Comparison with Similar Compounds
Similar Compounds
Amlodipine: Another dihydropyridine calcium antagonist with similar vasodilatory effects.
Nifedipine: Known for its rapid onset of action in treating hypertension.
Felodipine: Used for its long-acting effects in managing blood pressure.
Uniqueness
AMEP is unique due to its strong affinity for vascular smooth muscle over cardiac muscle, leading to fewer cardiac side effects. Its long-acting nature allows for less frequent dosing, making it a convenient option for patients with hypertension .
Properties
IUPAC Name |
aminomethylphosphonic acid | |
---|---|---|
Source | PubChem | |
URL | https://pubchem.ncbi.nlm.nih.gov | |
Description | Data deposited in or computed by PubChem | |
InChI |
InChI=1S/CH6NO3P/c2-1-6(3,4)5/h1-2H2,(H2,3,4,5) | |
Source | PubChem | |
URL | https://pubchem.ncbi.nlm.nih.gov | |
Description | Data deposited in or computed by PubChem | |
InChI Key |
MGRVRXRGTBOSHW-UHFFFAOYSA-N | |
Source | PubChem | |
URL | https://pubchem.ncbi.nlm.nih.gov | |
Description | Data deposited in or computed by PubChem | |
Canonical SMILES |
C(N)P(=O)(O)O | |
Source | PubChem | |
URL | https://pubchem.ncbi.nlm.nih.gov | |
Description | Data deposited in or computed by PubChem | |
Molecular Formula |
CH6NO3P | |
Source | PubChem | |
URL | https://pubchem.ncbi.nlm.nih.gov | |
Description | Data deposited in or computed by PubChem | |
DSSTOX Substance ID |
DTXSID5037490 | |
Record name | Aminomethylphosphonic acid | |
Source | EPA DSSTox | |
URL | https://comptox.epa.gov/dashboard/DTXSID5037490 | |
Description | DSSTox provides a high quality public chemistry resource for supporting improved predictive toxicology. | |
Molecular Weight |
111.04 g/mol | |
Source | PubChem | |
URL | https://pubchem.ncbi.nlm.nih.gov | |
Description | Data deposited in or computed by PubChem | |
CAS No. |
1066-51-9 | |
Record name | (Aminomethyl)phosphonic acid | |
Source | CAS Common Chemistry | |
URL | https://commonchemistry.cas.org/detail?cas_rn=1066-51-9 | |
Description | CAS Common Chemistry is an open community resource for accessing chemical information. Nearly 500,000 chemical substances from CAS REGISTRY cover areas of community interest, including common and frequently regulated chemicals, and those relevant to high school and undergraduate chemistry classes. This chemical information, curated by our expert scientists, is provided in alignment with our mission as a division of the American Chemical Society. | |
Explanation | The data from CAS Common Chemistry is provided under a CC-BY-NC 4.0 license, unless otherwise stated. | |
Record name | 1-Aminomethylphosphonic acid | |
Source | ChemIDplus | |
URL | https://pubchem.ncbi.nlm.nih.gov/substance/?source=chemidplus&sourceid=0001066519 | |
Description | ChemIDplus is a free, web search system that provides access to the structure and nomenclature authority files used for the identification of chemical substances cited in National Library of Medicine (NLM) databases, including the TOXNET system. | |
Record name | (Aminomethyl)phosphonic acid | |
Source | DTP/NCI | |
URL | https://dtp.cancer.gov/dtpstandard/servlet/dwindex?searchtype=NSC&outputformat=html&searchlist=30076 | |
Description | The NCI Development Therapeutics Program (DTP) provides services and resources to the academic and private-sector research communities worldwide to facilitate the discovery and development of new cancer therapeutic agents. | |
Explanation | Unless otherwise indicated, all text within NCI products is free of copyright and may be reused without our permission. Credit the National Cancer Institute as the source. | |
Record name | Aminomethylphosphonic acid | |
Source | EPA DSSTox | |
URL | https://comptox.epa.gov/dashboard/DTXSID5037490 | |
Description | DSSTox provides a high quality public chemistry resource for supporting improved predictive toxicology. | |
Record name | (Aminomethyl)phosphonic Acid | |
Source | European Chemicals Agency (ECHA) | |
URL | https://echa.europa.eu/information-on-chemicals | |
Description | The European Chemicals Agency (ECHA) is an agency of the European Union which is the driving force among regulatory authorities in implementing the EU's groundbreaking chemicals legislation for the benefit of human health and the environment as well as for innovation and competitiveness. | |
Explanation | Use of the information, documents and data from the ECHA website is subject to the terms and conditions of this Legal Notice, and subject to other binding limitations provided for under applicable law, the information, documents and data made available on the ECHA website may be reproduced, distributed and/or used, totally or in part, for non-commercial purposes provided that ECHA is acknowledged as the source: "Source: European Chemicals Agency, http://echa.europa.eu/". Such acknowledgement must be included in each copy of the material. ECHA permits and encourages organisations and individuals to create links to the ECHA website under the following cumulative conditions: Links can only be made to webpages that provide a link to the Legal Notice page. | |
Record name | AMINOMETHYLPHOSPHONIC ACID | |
Source | FDA Global Substance Registration System (GSRS) | |
URL | https://gsrs.ncats.nih.gov/ginas/app/beta/substances/90825O5C1U | |
Description | The FDA Global Substance Registration System (GSRS) enables the efficient and accurate exchange of information on what substances are in regulated products. Instead of relying on names, which vary across regulatory domains, countries, and regions, the GSRS knowledge base makes it possible for substances to be defined by standardized, scientific descriptions. | |
Explanation | Unless otherwise noted, the contents of the FDA website (www.fda.gov), both text and graphics, are not copyrighted. They are in the public domain and may be republished, reprinted and otherwise used freely by anyone without the need to obtain permission from FDA. Credit to the U.S. Food and Drug Administration as the source is appreciated but not required. | |
Retrosynthesis Analysis
AI-Powered Synthesis Planning: Our tool employs the Template_relevance Pistachio, Template_relevance Bkms_metabolic, Template_relevance Pistachio_ringbreaker, Template_relevance Reaxys, Template_relevance Reaxys_biocatalysis model, leveraging a vast database of chemical reactions to predict feasible synthetic routes.
One-Step Synthesis Focus: Specifically designed for one-step synthesis, it provides concise and direct routes for your target compounds, streamlining the synthesis process.
Accurate Predictions: Utilizing the extensive PISTACHIO, BKMS_METABOLIC, PISTACHIO_RINGBREAKER, REAXYS, REAXYS_BIOCATALYSIS database, our tool offers high-accuracy predictions, reflecting the latest in chemical research and data.
Strategy Settings
Precursor scoring | Relevance Heuristic |
---|---|
Min. plausibility | 0.01 |
Model | Template_relevance |
Template Set | Pistachio/Bkms_metabolic/Pistachio_ringbreaker/Reaxys/Reaxys_biocatalysis |
Top-N result to add to graph | 6 |
Feasible Synthetic Routes
Q1: What is the molecular formula and weight of (aminomethyl)phosphonic acid?
A: this compound has a molecular formula of CH6NO3P and a molecular weight of 111.04 g/mol. []
Q2: How is this compound typically characterized?
A: Various spectroscopic techniques are employed for its characterization, including FTIR, Raman, XPS, AFM, TEM, XRD, CHN, and UV analyses. [] Gas chromatography-mass spectrometry (GC-MS) is commonly used to analyze AMPA after derivatization with reagents like BSTFA + 10% TMCS. [, , ] Liquid chromatography with fluorescence detection (LC-FLD) is another widely used technique for analyzing AMPA in various matrices, often involving pre-column derivatization. [, , , ] Additionally, electrochemical techniques like differential pulse polarography have been explored for AMPA determination. []
Q3: What are some challenges in the analytical detection of this compound?
A: One challenge is the instability of derivatized AMPA, requiring analysis within a specific timeframe, ideally less than 24 hours. [] Achieving low limits of detection, especially in complex matrices like soil or food, can also be challenging, sometimes requiring extensive sample clean-up procedures. [, ]
Q4: Does this compound pose any environmental risks?
A: While considered less toxic than glyphosate, AMPA can contribute to eutrophication in aquatic ecosystems due to its phosphorus content. [] Its accumulation in biofilms raises concerns about potential impacts on aquatic food webs. [] Further research is necessary to fully understand its long-term ecological consequences.
Q5: Can this compound act as a chelating agent?
A: Yes, AMPA can act as a chelating agent. Studies have shown its ability to form five-membered chelate rings with various metal ions, including Mg2+, Ca2+, and transition metals. [] This property makes it useful in applications like the synthesis of metal-organic frameworks (MOFs). []
Q6: Are there any industrial applications for this compound?
A: Apart from its relevance to glyphosate, AMPA finds use in various fields. It serves as a starting material for synthesizing antibacterial phosphonopeptides that inhibit bacterial cell wall biosynthesis. [] It's also utilized in preparing functionalized graphene oxide for thorium(IV) ion adsorption, relevant in nuclear waste management. [] AMPA is also explored as a component in temperature-sensitive affinity chromatography materials for separating phosphoric acid compounds. [] Furthermore, its incorporation in perovskite solar cell fabrication enhances device efficiency and stability due to its strong anchoring abilities. []
Q7: What are the existing regulations surrounding this compound?
A: As the primary metabolite of glyphosate, AMPA is often monitored in conjunction with glyphosate in environmental and food samples. Regulations vary globally, but many countries have established maximum residue limits (MRLs) for glyphosate and AMPA in food and water. [, , ]
Disclaimer and Information on In-Vitro Research Products
Please be aware that all articles and product information presented on BenchChem are intended solely for informational purposes. The products available for purchase on BenchChem are specifically designed for in-vitro studies, which are conducted outside of living organisms. In-vitro studies, derived from the Latin term "in glass," involve experiments performed in controlled laboratory settings using cells or tissues. It is important to note that these products are not categorized as medicines or drugs, and they have not received approval from the FDA for the prevention, treatment, or cure of any medical condition, ailment, or disease. We must emphasize that any form of bodily introduction of these products into humans or animals is strictly prohibited by law. It is essential to adhere to these guidelines to ensure compliance with legal and ethical standards in research and experimentation.