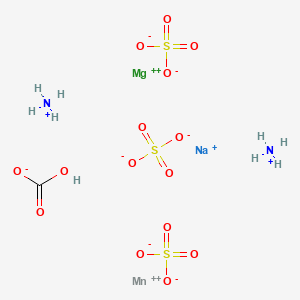
Carboxylin
- Click on QUICK INQUIRY to receive a quote from our team of experts.
- With the quality product at a COMPETITIVE price, you can focus more on your research.
Overview
Description
The compound “Carboxylin” is a multi-component chemical formulation used in various applications, including pharmaceuticals and industrial processes. Each component contributes unique properties, making the combination versatile and effective in different contexts.
Preparation Methods
Synthetic Routes and Reaction Conditions
-
Ammonium Sulfate: : Prepared by reacting ammonia with sulfuric acid.
Reaction: ( 2 \text{NH}_3 + \text{H}_2\text{SO}_4 \rightarrow (\text{NH}_4)_2\text{SO}_4 )
Conditions: Typically conducted at room temperature with controlled addition of ammonia to avoid excess heat.
-
Magnesium Sulfate: : Produced by reacting magnesium oxide or magnesium carbonate with sulfuric acid.
Reaction: ( \text{MgO} + \text{H}_2\text{SO}_4 \rightarrow \text{MgSO}_4 + \text{H}_2\text{O} )
Conditions: Requires heating to ensure complete reaction.
-
Manganese Sulfate: : Formed by treating manganese dioxide with sulfuric acid.
Reaction: ( \text{MnO}_2 + \text{H}_2\text{SO}_4 \rightarrow \text{MnSO}_4 + \text{H}_2\text{O} )
Conditions: Conducted at elevated temperatures to facilitate the reaction.
-
Sodium Bicarbonate: : Produced by the Solvay process, which involves the reaction of sodium chloride, ammonia, and carbon dioxide in water.
Reaction: ( \text{NaCl} + \text{NH}_3 + \text{CO}_2 + \text{H}_2\text{O} \rightarrow \text{NaHCO}_3 + \text{NH}_4\text{Cl} )
Conditions: Requires controlled temperature and pressure conditions.
-
Zinc Sulfate: : Prepared by reacting zinc oxide with sulfuric acid.
Reaction: ( \text{ZnO} + \text{H}_2\text{SO}_4 \rightarrow \text{ZnSO}_4 + \text{H}_2\text{O} )
Conditions: Typically conducted at room temperature with stirring.
Industrial Production Methods
The industrial production of this compound involves large-scale synthesis using the above reactions. The process includes:
Mixing: Combining the individual components in precise ratios.
Purification: Removing impurities through filtration and crystallization.
Drying: Ensuring the final product is free from excess moisture.
Chemical Reactions Analysis
Types of Reactions
Oxidation-Reduction: Manganese sulfate can undergo redox reactions, where manganese changes its oxidation state.
Substitution: Sodium bicarbonate can participate in substitution reactions, especially in acidic conditions.
Precipitation: Zinc sulfate can form precipitates with various anions.
Common Reagents and Conditions
Oxidizing Agents: Potassium permanganate for redox reactions involving manganese sulfate.
Acids: Hydrochloric acid for substitution reactions with sodium bicarbonate.
Bases: Sodium hydroxide for precipitation reactions with zinc sulfate.
Major Products
Manganese Dioxide: Formed from the oxidation of manganese sulfate.
Carbon Dioxide: Released during the decomposition of sodium bicarbonate.
Zinc Hydroxide: Precipitated from zinc sulfate in basic conditions.
Scientific Research Applications
Chemistry
Catalysis: Manganese compounds are used as catalysts in organic synthesis.
Buffer Solutions: Sodium bicarbonate is used to maintain pH in chemical reactions.
Biology
Nutrient Supplements: Magnesium and zinc are essential nutrients in biological systems.
Enzyme Activation: Manganese acts as a cofactor for various enzymes.
Medicine
Antacids: Sodium bicarbonate is used to neutralize stomach acid.
Electrolyte Balance: Magnesium sulfate is used in intravenous solutions to correct electrolyte imbalances.
Industry
Water Treatment: Zinc sulfate is used to control algae growth in water systems.
Fertilizers: Ammonium sulfate is a common nitrogen source in fertilizers.
Mechanism of Action
The compound exerts its effects through various mechanisms:
Ammonium Sulfate: Provides nitrogen for plant growth.
Magnesium Sulfate: Reduces muscle contractions by inhibiting calcium influx.
Manganese Sulfate: Acts as a cofactor for enzymes involved in metabolic processes.
Sodium Bicarbonate: Neutralizes acids by forming carbon dioxide and water.
Zinc Sulfate: Functions as a cofactor for numerous enzymes and supports immune function.
Comparison with Similar Compounds
Similar Compounds
Calcium Carbonate: Used as an antacid and calcium supplement.
Potassium Sulfate: Used in fertilizers and as a potassium supplement.
Copper Sulfate: Used in agriculture and as a fungicide.
Uniqueness
The combination of ammonium, magnesium, manganese, sodium bicarbonate, and zinc sulfate offers a unique blend of properties that are not found in single-component compounds. This combination provides a synergistic effect, enhancing the overall efficacy in various applications.
Properties
CAS No. |
37330-47-5 |
---|---|
Molecular Formula |
CH9MgMnN2NaO15S3 |
Molecular Weight |
487.5 g/mol |
IUPAC Name |
diazanium;magnesium;sodium;hydrogen carbonate;manganese(2+);trisulfate |
InChI |
InChI=1S/CH2O3.Mg.Mn.2H3N.Na.3H2O4S/c2-1(3)4;;;;;;3*1-5(2,3)4/h(H2,2,3,4);;;2*1H3;;3*(H2,1,2,3,4)/q;2*+2;;;+1;;;/p-5 |
InChI Key |
IUIYEXCXIIWZQN-UHFFFAOYSA-I |
SMILES |
C(=O)(O)[O-].[NH4+].[NH4+].[O-]S(=O)(=O)[O-].[O-]S(=O)(=O)[O-].[O-]S(=O)(=O)[O-].[Na+].[Mg+2].[Mn+2] |
Canonical SMILES |
C(=O)(O)[O-].[NH4+].[NH4+].[O-]S(=O)(=O)[O-].[O-]S(=O)(=O)[O-].[O-]S(=O)(=O)[O-].[Na+].[Mg+2].[Mn+2] |
37330-47-5 | |
Synonyms |
ammonium - magnesium - manganese - sodium bicarbonate - zinc sulfate ammonium, magnesium, manganese, sodium bicarbonate, zinc sulfate drug combination carboxylin carboxyline |
Origin of Product |
United States |
Disclaimer and Information on In-Vitro Research Products
Please be aware that all articles and product information presented on BenchChem are intended solely for informational purposes. The products available for purchase on BenchChem are specifically designed for in-vitro studies, which are conducted outside of living organisms. In-vitro studies, derived from the Latin term "in glass," involve experiments performed in controlled laboratory settings using cells or tissues. It is important to note that these products are not categorized as medicines or drugs, and they have not received approval from the FDA for the prevention, treatment, or cure of any medical condition, ailment, or disease. We must emphasize that any form of bodily introduction of these products into humans or animals is strictly prohibited by law. It is essential to adhere to these guidelines to ensure compliance with legal and ethical standards in research and experimentation.