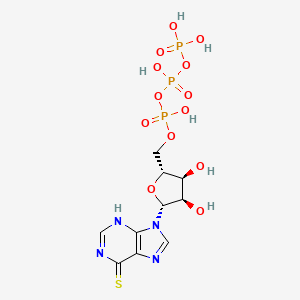
6-Mercaptopurine ribonucleoside triphosphate
Overview
Description
6-Mercaptopurine ribonucleoside triphosphate is an organic molecule with the chemical formula C10H15N4O13P3S. It is a derivative of 6-mercaptopurine, a well-known antimetabolite used in the treatment of leukemia and other autoimmune diseases . This compound is a ribonucleoside triphosphate, meaning it consists of a ribose sugar, a purine base (6-mercaptopurine), and three phosphate groups.
Preparation Methods
Synthetic Routes and Reaction Conditions: The synthesis of 6-mercaptopurine ribonucleoside triphosphate typically involves the phosphorylation of 6-mercaptopurine ribonucleoside. This can be achieved through a multi-step process:
Phosphorylation: The ribonucleoside is first phosphorylated using phosphorylating agents such as phosphorus oxychloride (POCl3) in the presence of a base like triethylamine.
Purification: The resulting product is purified using chromatographic techniques to isolate the triphosphate form.
Industrial Production Methods: Industrial production of this compound follows similar synthetic routes but on a larger scale. The process involves:
Bulk Synthesis: Large-scale phosphorylation reactions using automated reactors.
Purification: High-performance liquid chromatography (HPLC) or other advanced purification methods to ensure high purity and yield.
Chemical Reactions Analysis
Types of Reactions: 6-Mercaptopurine ribonucleoside triphosphate undergoes various chemical reactions, including:
Oxidation: It can be oxidized to form disulfides or sulfoxides.
Reduction: Reduction reactions can convert it back to its thiol form.
Substitution: Nucleophilic substitution reactions can occur at the purine base or the phosphate groups.
Common Reagents and Conditions:
Oxidation: Hydrogen peroxide (H2O2) or other oxidizing agents.
Reduction: Reducing agents like dithiothreitol (DTT).
Substitution: Nucleophiles such as amines or thiols under basic conditions.
Major Products:
Oxidation Products: Disulfides, sulfoxides.
Reduction Products: Thiol forms.
Substitution Products: Various substituted purine derivatives.
Scientific Research Applications
6-Mercaptopurine ribonucleoside triphosphate has several applications in scientific research:
Chemistry: Used as a model compound to study nucleotide analogs and their interactions.
Biology: Investigated for its role in cellular metabolism and its effects on nucleic acid synthesis.
Medicine: Explored for its potential in cancer therapy, particularly in leukemia treatment.
Industry: Utilized in the development of new pharmaceuticals and as a reference standard in analytical chemistry
Mechanism of Action
The mechanism of action of 6-mercaptopurine ribonucleoside triphosphate involves its incorporation into nucleic acids, where it acts as a purine antagonist. It competes with natural purine derivatives (hypoxanthine and guanine) for the enzyme hypoxanthine-guanine phosphoribosyltransferase (HGPRT). Once incorporated, it inhibits the synthesis of DNA and RNA, leading to cell cycle arrest and apoptosis in rapidly dividing cells .
Comparison with Similar Compounds
6-Thioguanine: Another thiopurine used in cancer therapy.
Azathioprine: A prodrug that is converted to 6-mercaptopurine in the body.
Thioinosine Monophosphate: A related nucleotide analog.
Uniqueness: 6-Mercaptopurine ribonucleoside triphosphate is unique due to its specific structure, which allows it to be incorporated into nucleic acids and exert its effects as a purine antagonist. Its triphosphate form enhances its ability to participate in biochemical reactions compared to its monophosphate or free base forms .
Biological Activity
6-Mercaptopurine ribonucleoside triphosphate (6-MP-RTP) is a nucleoside analog derived from 6-mercaptopurine (6-MP), a medication primarily used in the treatment of leukemia and autoimmune diseases. As a purine analog, 6-MP-RTP plays a crucial role in inhibiting nucleic acid synthesis, which is essential for cellular proliferation. This article explores the biological activity of 6-MP-RTP, focusing on its mechanisms of action, pharmacological effects, and relevant case studies.
6-MP-RTP exerts its biological effects primarily through the following mechanisms:
- Inhibition of Purine Synthesis : 6-MP is converted into various metabolites, including 6-MP-RTP, which inhibits enzymes involved in purine metabolism, such as amidophosphoribosyltransferase. This inhibition leads to reduced levels of purine nucleotides, ultimately affecting DNA and RNA synthesis.
- Incorporation into Nucleic Acids : As a triphosphate form, 6-MP-RTP can be incorporated into RNA and DNA. This incorporation can lead to the formation of faulty nucleic acids, disrupting normal cellular function and promoting apoptosis in rapidly dividing cells.
- Immunosuppressive Effects : The compound also exhibits immunosuppressive properties by affecting T-lymphocyte proliferation and cytokine production, making it useful in treating autoimmune conditions.
Biological Activity Data
The biological activity of 6-MP-RTP can be summarized in the following table:
Biological Activity | Effect | Reference |
---|---|---|
Inhibition of DNA synthesis | Decreased proliferation of cancer cells | |
RNA incorporation | Formation of dysfunctional RNA | |
Immunosuppression | Reduced T-cell activation | |
Cytotoxicity in leukemia cells | Induction of apoptosis | |
Synergistic effects with other drugs | Enhanced efficacy in combination therapies |
Case Study 1: Treatment of Acute Lymphoblastic Leukemia (ALL)
A clinical study investigated the efficacy of 6-MP in combination with other chemotherapeutic agents in children with ALL. The results demonstrated that patients receiving 6-MP exhibited higher remission rates compared to those on standard therapy alone. The study highlighted the role of 6-MP-RTP in enhancing the cytotoxic effects on leukemic cells while minimizing toxicity to normal cells.
Case Study 2: Autoimmune Disorders
In a cohort study involving patients with autoimmune diseases such as Crohn's disease and ulcerative colitis, treatment with 6-MP led to significant clinical improvement. The immunosuppressive effects mediated by 6-MP-RTP contributed to decreased inflammation and remission rates in these patients. Monitoring of lymphocyte counts indicated a dose-dependent relationship between 6-MP administration and immunosuppression.
Research Findings
Recent research has further elucidated the biological activity of 6-MP-RTP:
- Metabolic Pathways : A study identified key metabolic pathways involved in the conversion of 6-MP to its active forms, including 6-MP-RTP. Understanding these pathways is crucial for optimizing therapeutic strategies and minimizing side effects .
- Pharmacogenomics : Variations in genes related to thiopurine metabolism, such as TPMT (thiopurine S-methyltransferase), significantly affect the efficacy and toxicity profiles of 6-MP treatments. Genotyping patients for TPMT variants can help tailor dosing regimens for improved outcomes .
- Combination Therapies : Investigations into combination therapies involving 6-MP-RTP have shown promising results in enhancing treatment efficacy for various cancers. For instance, combining 6-MP with other agents like methotrexate has been shown to produce synergistic effects, leading to improved patient outcomes .
Properties
IUPAC Name |
[[(2R,3S,4R,5R)-3,4-dihydroxy-5-(6-sulfanylidene-3H-purin-9-yl)oxolan-2-yl]methoxy-hydroxyphosphoryl] phosphono hydrogen phosphate | |
---|---|---|
Source | PubChem | |
URL | https://pubchem.ncbi.nlm.nih.gov | |
Description | Data deposited in or computed by PubChem | |
InChI |
InChI=1S/C10H15N4O13P3S/c15-6-4(1-24-29(20,21)27-30(22,23)26-28(17,18)19)25-10(7(6)16)14-3-13-5-8(14)11-2-12-9(5)31/h2-4,6-7,10,15-16H,1H2,(H,20,21)(H,22,23)(H,11,12,31)(H2,17,18,19)/t4-,6-,7-,10-/m1/s1 | |
Source | PubChem | |
URL | https://pubchem.ncbi.nlm.nih.gov | |
Description | Data deposited in or computed by PubChem | |
InChI Key |
GQNRDWAOABGWGP-KQYNXXCUSA-N | |
Source | PubChem | |
URL | https://pubchem.ncbi.nlm.nih.gov | |
Description | Data deposited in or computed by PubChem | |
Canonical SMILES |
C1=NC(=S)C2=C(N1)N(C=N2)C3C(C(C(O3)COP(=O)(O)OP(=O)(O)OP(=O)(O)O)O)O | |
Source | PubChem | |
URL | https://pubchem.ncbi.nlm.nih.gov | |
Description | Data deposited in or computed by PubChem | |
Isomeric SMILES |
C1=NC(=S)C2=C(N1)N(C=N2)[C@H]3[C@@H]([C@@H]([C@H](O3)COP(=O)(O)OP(=O)(O)OP(=O)(O)O)O)O | |
Source | PubChem | |
URL | https://pubchem.ncbi.nlm.nih.gov | |
Description | Data deposited in or computed by PubChem | |
Molecular Formula |
C10H15N4O13P3S | |
Source | PubChem | |
URL | https://pubchem.ncbi.nlm.nih.gov | |
Description | Data deposited in or computed by PubChem | |
Molecular Weight |
524.23 g/mol | |
Source | PubChem | |
URL | https://pubchem.ncbi.nlm.nih.gov | |
Description | Data deposited in or computed by PubChem | |
CAS No. |
27652-34-2 | |
Record name | Thio-ITP | |
Source | CAS Common Chemistry | |
URL | https://commonchemistry.cas.org/detail?cas_rn=27652-34-2 | |
Description | CAS Common Chemistry is an open community resource for accessing chemical information. Nearly 500,000 chemical substances from CAS REGISTRY cover areas of community interest, including common and frequently regulated chemicals, and those relevant to high school and undergraduate chemistry classes. This chemical information, curated by our expert scientists, is provided in alignment with our mission as a division of the American Chemical Society. | |
Explanation | The data from CAS Common Chemistry is provided under a CC-BY-NC 4.0 license, unless otherwise stated. | |
Record name | 6-Thioinosine-5'-triphosphate | |
Source | ChemIDplus | |
URL | https://pubchem.ncbi.nlm.nih.gov/substance/?source=chemidplus&sourceid=0027652342 | |
Description | ChemIDplus is a free, web search system that provides access to the structure and nomenclature authority files used for the identification of chemical substances cited in National Library of Medicine (NLM) databases, including the TOXNET system. | |
Retrosynthesis Analysis
AI-Powered Synthesis Planning: Our tool employs the Template_relevance Pistachio, Template_relevance Bkms_metabolic, Template_relevance Pistachio_ringbreaker, Template_relevance Reaxys, Template_relevance Reaxys_biocatalysis model, leveraging a vast database of chemical reactions to predict feasible synthetic routes.
One-Step Synthesis Focus: Specifically designed for one-step synthesis, it provides concise and direct routes for your target compounds, streamlining the synthesis process.
Accurate Predictions: Utilizing the extensive PISTACHIO, BKMS_METABOLIC, PISTACHIO_RINGBREAKER, REAXYS, REAXYS_BIOCATALYSIS database, our tool offers high-accuracy predictions, reflecting the latest in chemical research and data.
Strategy Settings
Precursor scoring | Relevance Heuristic |
---|---|
Min. plausibility | 0.01 |
Model | Template_relevance |
Template Set | Pistachio/Bkms_metabolic/Pistachio_ringbreaker/Reaxys/Reaxys_biocatalysis |
Top-N result to add to graph | 6 |
Feasible Synthetic Routes
Q1: How does 6-Mercaptopurine ribonucleoside triphosphate (6-thio-ITP) interact with its target and what are the downstream effects?
A: 6-thio-ITP acts as a competitive inhibitor of RNA polymerase I and II, which are essential enzymes for RNA synthesis within cells []. Specifically, it competes with guanosine 5'-triphosphate (GTP) for binding to the enzyme's active site []. This competition disrupts the transcription process, ultimately leading to the inhibition of RNA synthesis. Since RNA synthesis is crucial for cell growth and survival, this inhibition contributes to the cytotoxic effect of 6-mercaptopurine, a known antitumor agent [].
Q2: Can 6-thio-ITP be utilized as a structural probe for studying enzymes like Escherichia coli glutamine synthetase?
A: Yes, research suggests that 6-thio-ITP, alongside its analog 8-mercaptoadenosine 5'-triphosphate (8-S-ATP), can serve as a structural probe for enzymes like Escherichia coli glutamine synthetase []. These compounds can substitute for ATP in the autoinactivation reaction of the enzyme []. Modifications with fluorescent and chromogenic probes or electron-dense markers allow for studying the enzyme's structure and interactions []. Furthermore, the spectral properties of 6-thio-ITP change upon binding to the enzyme, offering insights into the environment of the binding site [].
Disclaimer and Information on In-Vitro Research Products
Please be aware that all articles and product information presented on BenchChem are intended solely for informational purposes. The products available for purchase on BenchChem are specifically designed for in-vitro studies, which are conducted outside of living organisms. In-vitro studies, derived from the Latin term "in glass," involve experiments performed in controlled laboratory settings using cells or tissues. It is important to note that these products are not categorized as medicines or drugs, and they have not received approval from the FDA for the prevention, treatment, or cure of any medical condition, ailment, or disease. We must emphasize that any form of bodily introduction of these products into humans or animals is strictly prohibited by law. It is essential to adhere to these guidelines to ensure compliance with legal and ethical standards in research and experimentation.