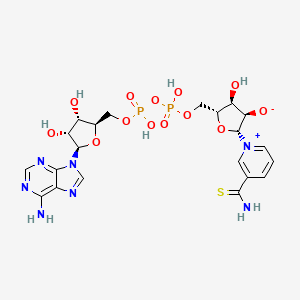
Thio-NAD
Overview
Description
Thio-Nicotinamide Adenine Dinucleotide (Thio-NAD) is a modified form of Nicotinamide Adenine Dinucleotide (NAD) where a sulfur atom replaces an oxygen atom in the pyrophosphate group. This modification imparts unique properties to this compound, making it more reactive and suitable for specific biochemical applications. This compound is primarily used in enzymatic reactions and diagnostic assays due to its enhanced absorbance properties at 405 nm, which is a commonly available wavelength on microplate readers .
Mechanism of Action
Target of Action
Thio-Nicotinamide Adenine Dinucleotide, also known as Thio-NAD, primarily targets enzymes that consume Nicotinamide Adenine Dinucleotide (NAD+). The use of this compound instead of NAD+ as a substrate for these enzymes is advantageous since the reduced form of this compound exhibits a substantial increase in absorbance at 405 nM . One of the key functions of this compound is its role in maintaining cellular energy levels. This compound serves as a coenzyme for the enzyme Nicotinamide Adenine Dinucleotide Phosphate (NADP) reductase, which is involved in the production of Adenosine Triphosphate (ATP), the primary energy currency of the cell .
Mode of Action
The mechanism of action of this compound involves its conversion to NAD+ within cells. Once inside the cell, this compound is broken down into NAD+ and a sulfur-containing compound. This process replenishes cellular NAD+ levels, allowing for increased activity of NAD±dependent enzymes and sirtuins . The sulfur atom in this compound increases the electron density in the molecule, making it more reactive than NAD. This increased reactivity enhances the ability of this compound to participate in redox reactions and serve as a cofactor for a variety of enzymes involved in cellular metabolism .
Biochemical Pathways
This compound plays a crucial role in redox reactions, which involve the transfer of electrons between molecules. It is involved in various energy production processes, such as the Krebs cycle, fatty acid oxidation, glycolysis, and serine biosynthesis . By facilitating the transfer of electrons during oxidative phosphorylation, this compound helps generate ATP, which is essential for various cellular processes, including muscle contraction, nerve transmission, and protein synthesis .
Pharmacokinetics
It is known that the bioavailability and stability of this compound can be influenced by various factors, including the method of administration and the presence of other substances in the body .
Result of Action
The unique properties of this compound make it a promising molecule for therapeutic interventions in various diseases. Research has shown that this compound supplementation can enhance cellular energy production, improve metabolic function, and protect cells from oxidative damage .
Action Environment
The action, efficacy, and stability of this compound can be influenced by various environmental factors. For instance, the presence of other substances in the body, the pH of the environment, and the temperature can all affect the activity of this compound
Biochemical Analysis
Biochemical Properties
Thio-NAD plays a crucial role in biochemical reactions, particularly in redox reactions where it acts as an electron carrier. It interacts with various enzymes, proteins, and other biomolecules. For instance, this compound is involved in the activity of soluble pyridine nucleotide transhydrogenase from Escherichia coli, where it serves as a substrate for hydride transfer reactions . The enzyme catalyzes the reversible transfer of hydride ions between NAD(H) and NADP(H), maintaining the redox balance within the cell. This compound’s interaction with this enzyme highlights its importance in cellular redox homeostasis.
Cellular Effects
This compound influences various cellular processes, including cell signaling pathways, gene expression, and cellular metabolism. It has been observed that this compound can affect the activity of enzymes involved in metabolic pathways, thereby altering the metabolic flux within cells . Additionally, this compound’s role in redox reactions can impact the cellular redox state, influencing processes such as oxidative stress response and apoptosis.
Molecular Mechanism
At the molecular level, this compound exerts its effects through binding interactions with specific biomolecules. For example, it binds to the active site of soluble pyridine nucleotide transhydrogenase, facilitating the transfer of hydride ions . This binding interaction is crucial for the enzyme’s catalytic activity. This compound can also act as an inhibitor or activator of other enzymes, depending on the context of the reaction and the specific enzyme involved.
Temporal Effects in Laboratory Settings
In laboratory settings, the effects of this compound can change over time. Its stability and degradation are important factors to consider. This compound is relatively stable under standard laboratory conditions, but its long-term effects on cellular function can vary. Studies have shown that this compound can maintain its activity for extended periods, although its efficacy may decrease over time due to potential degradation .
Dosage Effects in Animal Models
The effects of this compound can vary with different dosages in animal models. At lower doses, this compound may enhance certain metabolic processes without causing adverse effects. At higher doses, it may exhibit toxic effects or disrupt normal cellular functions. It is essential to determine the optimal dosage to achieve the desired effects while minimizing potential toxicity .
Metabolic Pathways
This compound is involved in several metabolic pathways, particularly those related to redox reactions. It interacts with enzymes such as soluble pyridine nucleotide transhydrogenase, which plays a role in maintaining the balance between NAD(H) and NADP(H) within the cell . This compound’s involvement in these pathways can influence metabolic flux and the levels of various metabolites.
Transport and Distribution
Within cells and tissues, this compound is transported and distributed through specific transporters and binding proteins. These transporters facilitate the movement of this compound across cellular membranes, ensuring its availability for biochemical reactions. The distribution of this compound within cells can affect its localization and accumulation, influencing its overall activity and function .
Subcellular Localization
This compound’s subcellular localization is determined by targeting signals and post-translational modifications that direct it to specific compartments or organelles. Its localization can impact its activity and function, as it may interact with different biomolecules depending on its location within the cell. Understanding the subcellular distribution of this compound is crucial for elucidating its role in cellular processes .
Preparation Methods
Synthetic Routes and Reaction Conditions: Thio-NAD is synthesized enzymatically by incorporating a sulfur atom into the NAD molecule. The synthesis involves the use of specific enzymes that facilitate the incorporation of sulfur into the pyrophosphate group of NAD. The reaction conditions typically include a controlled environment with specific pH, temperature, and substrate concentrations to ensure the efficient production of this compound .
Industrial Production Methods: In industrial settings, this compound is produced using large-scale enzymatic reactors. The process involves the continuous feeding of substrates and enzymes into the reactor, maintaining optimal conditions for the enzymatic reaction. The product is then purified using chromatographic techniques to achieve high purity and concentration .
Chemical Reactions Analysis
Types of Reactions: Thio-NAD undergoes various chemical reactions, including oxidation, reduction, and substitution. The sulfur atom in this compound increases its reactivity, making it a suitable cofactor for redox reactions.
Common Reagents and Conditions:
Oxidation: this compound can be oxidized to its oxidized form using oxidizing agents such as hydrogen peroxide or molecular oxygen.
Reduction: this compound can be reduced to its reduced form using reducing agents such as sodium borohydride or dithiothreitol.
Substitution: this compound can undergo substitution reactions where the sulfur atom can be replaced by other nucleophiles under specific conditions.
Major Products: The major products formed from these reactions include the oxidized and reduced forms of this compound, as well as substituted derivatives depending on the nucleophiles used in the reactions .
Scientific Research Applications
Thio-NAD has a wide range of applications in scientific research, including:
Chemistry: this compound is used as a cofactor in various enzymatic reactions to study reaction kinetics and mechanisms.
Biology: this compound is used in biochemical assays to detect and quantify specific proteins and metabolites.
Medicine: this compound is used in diagnostic assays for detecting diseases such as COVID-19 and cancer. .
Industry: this compound is used in industrial bioprocesses to monitor and optimize enzymatic reactions.
Comparison with Similar Compounds
Nicotinamide Adenine Dinucleotide (NAD): The parent compound of Thio-NAD, involved in redox reactions in cellular metabolism.
Nicotinamide Adenine Dinucleotide Phosphate (NADP): A phosphorylated form of NAD, involved in anabolic reactions.
Flavin Adenine Dinucleotide (FAD): Another coenzyme involved in redox reactions, but with a different structure and function.
Uniqueness of this compound: this compound is unique due to the presence of a sulfur atom in its structure, which increases its reactivity and absorbance properties. This makes this compound more suitable for specific biochemical applications, such as ultrasensitive diagnostic assays and enzymatic reactions that require high reactivity .
This compound’s unique properties and wide range of applications make it a valuable compound in scientific research and industrial processes. Its enhanced reactivity and absorbance properties provide significant advantages over similar compounds, making it an essential tool in various fields of study.
Properties
CAS No. |
4090-29-3 |
---|---|
Molecular Formula |
C21H27N7O13P2S |
Molecular Weight |
679.5 g/mol |
IUPAC Name |
[[(2R,3S,4R,5R)-5-(6-aminopurin-9-yl)-3,4-dihydroxyoxolan-2-yl]methoxy-hydroxyphosphoryl] [(2R,3S,4R,5R)-5-(3-carbamothioylpyridin-1-ium-1-yl)-3,4-dihydroxyoxolan-2-yl]methyl phosphate |
InChI |
InChI=1S/C21H27N7O13P2S/c22-17-12-19(25-7-24-17)28(8-26-12)21-16(32)14(30)11(40-21)6-38-43(35,36)41-42(33,34)37-5-10-13(29)15(31)20(39-10)27-3-1-2-9(4-27)18(23)44/h1-4,7-8,10-11,13-16,20-21,29-32H,5-6H2,(H5-,22,23,24,25,33,34,35,36,44)/t10-,11-,13-,14-,15-,16-,20-,21-/m1/s1 |
InChI Key |
UQYPZLRUJKCREN-NNYOXOHSSA-N |
Isomeric SMILES |
C1=CC(=C[N+](=C1)[C@H]2[C@@H]([C@@H]([C@H](O2)COP(=O)([O-])OP(=O)(O)OC[C@@H]3[C@H]([C@H]([C@@H](O3)N4C=NC5=C(N=CN=C54)N)O)O)O)O)C(=S)N |
SMILES |
C1=CC(=C[N+](=C1)C2C(C(C(O2)COP(=O)(O)OP(=O)(O)OCC3C(C(C(O3)N4C=NC5=C(N=CN=C54)N)O)O)O)[O-])C(=S)N |
Canonical SMILES |
C1=CC(=C[N+](=C1)C2C(C(C(O2)COP(=O)([O-])OP(=O)(O)OCC3C(C(C(O3)N4C=NC5=C(N=CN=C54)N)O)O)O)O)C(=S)N |
Synonyms |
thio-NAD thionicotinamide adenine dinucleotide TNAD(+) |
Origin of Product |
United States |
Retrosynthesis Analysis
AI-Powered Synthesis Planning: Our tool employs the Template_relevance Pistachio, Template_relevance Bkms_metabolic, Template_relevance Pistachio_ringbreaker, Template_relevance Reaxys, Template_relevance Reaxys_biocatalysis model, leveraging a vast database of chemical reactions to predict feasible synthetic routes.
One-Step Synthesis Focus: Specifically designed for one-step synthesis, it provides concise and direct routes for your target compounds, streamlining the synthesis process.
Accurate Predictions: Utilizing the extensive PISTACHIO, BKMS_METABOLIC, PISTACHIO_RINGBREAKER, REAXYS, REAXYS_BIOCATALYSIS database, our tool offers high-accuracy predictions, reflecting the latest in chemical research and data.
Strategy Settings
Precursor scoring | Relevance Heuristic |
---|---|
Min. plausibility | 0.01 |
Model | Template_relevance |
Template Set | Pistachio/Bkms_metabolic/Pistachio_ringbreaker/Reaxys/Reaxys_biocatalysis |
Top-N result to add to graph | 6 |
Feasible Synthetic Routes
A: Thio-NAD is structurally similar to NAD+ but contains a sulfur atom replacing the oxygen atom in the carboxyamide group of the nicotinamide ring. This seemingly small modification can significantly impact its binding affinity and reactivity with various enzymes. [, ]
A: The sulfur substitution can alter the twist of the 3-carbothiamide group relative to the pyridine ring, affecting its interaction with enzymes. While Thio-NADP+ remains a good substrate for some enzymes like transhydrogenase, this compound+ often displays weaker binding and slower reaction rates compared to NAD+. This suggests that the sulfur atom might hinder conformational changes necessary for optimal substrate binding and catalysis. [, ]
A: Studies with transhydrogenase, an enzyme coupling NADH oxidation to NADP+ reduction, revealed that while Thio-NADP(H) acts as a good analogue for NADP(H), this compound(H) poorly mimics NAD(H). This difference stems from the altered conformational changes induced by this compound(H) impacting the hydride transfer process. []
ANone: The molecular formula of this compound+ is C21H27N7O13P2S, and its molecular weight is 663.49 g/mol.
A: Thio-NADH exhibits a distinct absorption maximum at 400 nm, making it easily distinguishable from NADH, which absorbs at 340 nm. This spectral shift allows for direct measurement of Thio-NADH accumulation in enzymatic assays. [, , , ]
A: Research suggests this compound demonstrates good stability in various buffer systems and temperatures, making it suitable for a wide range of biochemical and enzymatic assays. [, ]
A: Due to its distinct spectral properties and compatibility with various enzymes, this compound has found applications in developing highly sensitive enzymatic assays for detecting low concentrations of various analytes like proteins, cholesterol, and mevalonic acid. [, , , ]
A: this compound has been successfully employed in enzymatic assays utilizing enzymes like 3α-hydroxysteroid dehydrogenase (3α-HSD), glucose dehydrogenase, carnitine dehydrogenase, and hydroxybutyrate dehydrogenase. [, , , , ]
A: this compound's utility in ultrasensitive assays stems from its ability to participate in enzyme cycling reactions. In these reactions, a target analyte triggers a cascade where this compound is continuously reduced to Thio-NADH. The accumulating Thio-NADH, easily quantifiable at 400 nm, serves as a amplified signal proportional to the initial analyte concentration. [, , , ]
A: While the provided research primarily focuses on experimental studies, computational approaches like molecular docking and molecular dynamics simulations can offer valuable insights into the binding mode, affinity, and conformational changes upon this compound interaction with various enzymes. []
A: The sulfur substitution in this compound represents a prime example of structure-activity relationship studies. This modification significantly alters its interaction with certain enzymes, highlighting the importance of the carboxyamide group for optimal binding and catalysis. [, , ]
A: Developing stable formulations of this compound and optimizing its storage conditions are crucial for broader research and potential therapeutic applications. Encapsulation techniques and novel excipients could be explored to enhance its stability, solubility, and bioavailability. [, ]
A: While not explicitly addressed in the provided research, comprehensive safety and toxicological studies are crucial before considering this compound for therapeutic applications. Adhering to relevant regulatory guidelines and ensuring responsible handling practices are paramount throughout its development and application. []
Disclaimer and Information on In-Vitro Research Products
Please be aware that all articles and product information presented on BenchChem are intended solely for informational purposes. The products available for purchase on BenchChem are specifically designed for in-vitro studies, which are conducted outside of living organisms. In-vitro studies, derived from the Latin term "in glass," involve experiments performed in controlled laboratory settings using cells or tissues. It is important to note that these products are not categorized as medicines or drugs, and they have not received approval from the FDA for the prevention, treatment, or cure of any medical condition, ailment, or disease. We must emphasize that any form of bodily introduction of these products into humans or animals is strictly prohibited by law. It is essential to adhere to these guidelines to ensure compliance with legal and ethical standards in research and experimentation.