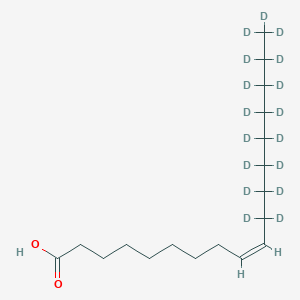
Oleic Acid-d17
Overview
Description
Oleic Acid-d17 is a deuterated form of oleic acid, a monounsaturated fatty acid. The deuterium atoms replace hydrogen atoms in the molecule, making it useful as an internal standard in mass spectrometry and other analytical techniques. This compound is often used in research to study lipid metabolism and the behavior of fatty acids in biological systems.
Mechanism of Action
Target of Action
Oleic Acid-d17, a deuterium-labeled form of Oleic Acid , primarily targets Na+/K+ ATPase . This enzyme plays a crucial role in maintaining the electrochemical gradient across the cell membrane, which is vital for numerous physiological processes .
Mode of Action
This compound interacts with its target, Na+/K+ ATPase, by acting as an activator . This interaction enhances the enzyme’s activity, promoting the exchange of sodium and potassium ions across the cell membrane .
Biochemical Pathways
This compound influences several biochemical pathways. It upregulates the expression of genes involved in fatty acid oxidation (FAO) via the deacetylation of PGC1α, a process facilitated by the PKA-dependent activation of the SIRT1-PGC1α complex . This leads to enhanced FAO, contributing to energy production and lipid metabolism .
Pharmacokinetics
Deuterium substitution in drug molecules, like in this compound, has been noted to potentially affect the pharmacokinetic and metabolic profiles of drugs .
Result of Action
The activation of Na+/K+ ATPase by this compound impacts various cellular processes. For instance, it inhibits collagen-stimulated platelet aggregation and fMLF-induced neutrophil aggregation and degranulation . Additionally, it induces the release of intracellular calcium in human platelets . In vivo, this compound increases the production of inflammatory mediators such as TNF-α, IL-8, IL-6, and IL-1β, and promotes neutrophil accumulation and cell death .
Biochemical Analysis
Biochemical Properties
Oleic Acid-d17 plays a significant role in various biochemical reactions. It interacts with several enzymes, proteins, and other biomolecules. One of the key enzymes it interacts with is stearoyl-CoA desaturase, which converts stearic acid to oleic acid. This interaction is crucial for maintaining the balance of saturated and unsaturated fatty acids in the body. Additionally, this compound is involved in the regulation of low-density lipoprotein (LDL) receptors, influencing cholesterol metabolism .
Cellular Effects
This compound has profound effects on various cell types and cellular processes. It influences cell function by modulating cell signaling pathways, gene expression, and cellular metabolism. For instance, this compound has been shown to increase the number of lipid droplets in cells, which are essential for storing and metabolizing fats. It also protects cellular membranes from oxidative damage, thereby enhancing cell viability and longevity . Furthermore, this compound can regulate the expression of genes involved in fatty acid oxidation and inflammation, impacting overall cellular health .
Molecular Mechanism
At the molecular level, this compound exerts its effects through several mechanisms. It binds to and activates peroxisome proliferator-activated receptors (PPARs), which are nuclear receptors that regulate gene expression related to lipid metabolism and inflammation. This compound also modulates the activity of enzymes such as acetyl-CoA carboxylase and fatty acid synthase, which are involved in fatty acid synthesis and degradation . Additionally, it influences the deacetylation of PGC1α by PKA-dependent activation of the SIRT1-PGC1α complex, promoting fatty acid oxidation .
Temporal Effects in Laboratory Settings
In laboratory settings, the effects of this compound can change over time. Studies have shown that its stability and degradation can impact its long-term effects on cellular function. For example, this compound has been observed to maintain its protective effects on cellular membranes for extended periods, but its efficacy may decrease over time due to degradation . Long-term studies have also indicated that this compound can influence cellular metabolism and gene expression over prolonged exposure .
Dosage Effects in Animal Models
The effects of this compound vary with different dosages in animal models. At lower doses, it has been shown to have beneficial effects on lipid metabolism and inflammation. At higher doses, this compound can exhibit toxic or adverse effects, such as inducing oxidative stress and inflammation . Threshold effects have been observed, where a specific dosage range is required to achieve the desired therapeutic effects without causing harm .
Metabolic Pathways
This compound is involved in several metabolic pathways, including fatty acid oxidation and synthesis. It interacts with enzymes such as stearoyl-CoA desaturase and acetyl-CoA carboxylase, which play crucial roles in these pathways. This compound also affects metabolic flux by regulating the levels of key metabolites, such as acetyl-CoA and malonyl-CoA . These interactions are essential for maintaining energy balance and lipid homeostasis in cells .
Transport and Distribution
Within cells and tissues, this compound is transported and distributed through various mechanisms. It interacts with transporters and binding proteins, such as fatty acid-binding proteins (FABPs), which facilitate its movement across cellular membranes. This compound can also accumulate in specific cellular compartments, such as lipid droplets and mitochondria, where it exerts its metabolic effects .
Subcellular Localization
This compound is localized in several subcellular compartments, including the endoplasmic reticulum, mitochondria, and lipid droplets. Its activity and function can be influenced by its localization. For example, in the endoplasmic reticulum, this compound is involved in the synthesis of phospholipids and triglycerides. In mitochondria, it plays a role in fatty acid oxidation and energy production . Targeting signals and post-translational modifications can direct this compound to specific compartments, enhancing its functional specificity .
Preparation Methods
Synthetic Routes and Reaction Conditions
Oleic Acid-d17 is synthesized by incorporating deuterium into oleic acid. This can be achieved through catalytic hydrogenation using deuterium gas. The reaction typically involves the use of a palladium or platinum catalyst under controlled conditions to ensure the selective incorporation of deuterium atoms.
Industrial Production Methods
In an industrial setting, the production of this compound involves the hydrogenation of oleic acid in the presence of deuterium gas. The process is carried out in large reactors with precise control over temperature and pressure to maximize the yield and purity of the deuterated product.
Chemical Reactions Analysis
Types of Reactions
Oleic Acid-d17 undergoes various chemical reactions, including:
Oxidation: this compound can be oxidized to form a variety of products, including aldehydes, ketones, and carboxylic acids.
Reduction: The compound can be reduced to form saturated fatty acids.
Substitution: Deuterium atoms in this compound can be substituted with other atoms or groups under specific conditions.
Common Reagents and Conditions
Oxidation: Common oxidizing agents include potassium permanganate and ozone. The reactions are typically carried out under mild conditions to prevent over-oxidation.
Reduction: Hydrogen gas in the presence of a metal catalyst such as palladium or platinum is commonly used for reduction reactions.
Substitution: Various reagents, including halogens and organometallic compounds, can be used for substitution reactions.
Major Products Formed
Oxidation: Products include nonanoic acid and azelaic acid.
Reduction: The major product is stearic acid.
Substitution: Depending on the substituent, products can vary widely, including halogenated fatty acids and other derivatives.
Scientific Research Applications
Oleic Acid-d17 has a wide range of applications in scientific research:
Chemistry: Used as an internal standard in mass spectrometry for the quantification of fatty acids.
Biology: Helps in studying lipid metabolism and the role of fatty acids in cellular processes.
Medicine: Used in research on metabolic disorders, including diabetes and obesity.
Industry: Employed in the development of new materials and products, including cosmetics and pharmaceuticals.
Properties
IUPAC Name |
(Z)-11,11,12,12,13,13,14,14,15,15,16,16,17,17,18,18,18-heptadecadeuteriooctadec-9-enoic acid | |
---|---|---|
Source | PubChem | |
URL | https://pubchem.ncbi.nlm.nih.gov | |
Description | Data deposited in or computed by PubChem | |
InChI |
InChI=1S/C18H34O2/c1-2-3-4-5-6-7-8-9-10-11-12-13-14-15-16-17-18(19)20/h9-10H,2-8,11-17H2,1H3,(H,19,20)/b10-9-/i1D3,2D2,3D2,4D2,5D2,6D2,7D2,8D2 | |
Source | PubChem | |
URL | https://pubchem.ncbi.nlm.nih.gov | |
Description | Data deposited in or computed by PubChem | |
InChI Key |
ZQPPMHVWECSIRJ-DUGYPAGXSA-N | |
Source | PubChem | |
URL | https://pubchem.ncbi.nlm.nih.gov | |
Description | Data deposited in or computed by PubChem | |
Canonical SMILES |
CCCCCCCCC=CCCCCCCCC(=O)O | |
Source | PubChem | |
URL | https://pubchem.ncbi.nlm.nih.gov | |
Description | Data deposited in or computed by PubChem | |
Isomeric SMILES |
[2H]C([2H])([2H])C([2H])([2H])C([2H])([2H])C([2H])([2H])C([2H])([2H])C([2H])([2H])C([2H])([2H])C([2H])([2H])/C=C\CCCCCCCC(=O)O | |
Source | PubChem | |
URL | https://pubchem.ncbi.nlm.nih.gov | |
Description | Data deposited in or computed by PubChem | |
Molecular Formula |
C18H34O2 | |
Source | PubChem | |
URL | https://pubchem.ncbi.nlm.nih.gov | |
Description | Data deposited in or computed by PubChem | |
DSSTOX Substance ID |
DTXSID70616430 | |
Record name | (9Z)-(11,11,12,12,13,13,14,14,15,15,16,16,17,17,18,18,18-~2~H_17_)Octadec-9-enoic acid | |
Source | EPA DSSTox | |
URL | https://comptox.epa.gov/dashboard/DTXSID70616430 | |
Description | DSSTox provides a high quality public chemistry resource for supporting improved predictive toxicology. | |
Molecular Weight |
299.6 g/mol | |
Source | PubChem | |
URL | https://pubchem.ncbi.nlm.nih.gov | |
Description | Data deposited in or computed by PubChem | |
CAS No. |
223487-44-3 | |
Record name | (9Z)-(11,11,12,12,13,13,14,14,15,15,16,16,17,17,18,18,18-~2~H_17_)Octadec-9-enoic acid | |
Source | EPA DSSTox | |
URL | https://comptox.epa.gov/dashboard/DTXSID70616430 | |
Description | DSSTox provides a high quality public chemistry resource for supporting improved predictive toxicology. | |
Retrosynthesis Analysis
AI-Powered Synthesis Planning: Our tool employs the Template_relevance Pistachio, Template_relevance Bkms_metabolic, Template_relevance Pistachio_ringbreaker, Template_relevance Reaxys, Template_relevance Reaxys_biocatalysis model, leveraging a vast database of chemical reactions to predict feasible synthetic routes.
One-Step Synthesis Focus: Specifically designed for one-step synthesis, it provides concise and direct routes for your target compounds, streamlining the synthesis process.
Accurate Predictions: Utilizing the extensive PISTACHIO, BKMS_METABOLIC, PISTACHIO_RINGBREAKER, REAXYS, REAXYS_BIOCATALYSIS database, our tool offers high-accuracy predictions, reflecting the latest in chemical research and data.
Strategy Settings
Precursor scoring | Relevance Heuristic |
---|---|
Min. plausibility | 0.01 |
Model | Template_relevance |
Template Set | Pistachio/Bkms_metabolic/Pistachio_ringbreaker/Reaxys/Reaxys_biocatalysis |
Top-N result to add to graph | 6 |
Feasible Synthetic Routes
Q1: Why is Oleic Acid-d17 used in the research paper instead of regular Oleic Acid?
A1: this compound is a deuterated form of Oleic Acid, meaning it has a slightly different mass due to the presence of heavier hydrogen isotopes (deuterium). This mass difference allows it to be easily distinguishable from naturally occurring Oleic Acid when analyzed using mass spectrometry. In the research paper, this compound serves as an internal standard []. Internal standards are crucial in quantitative analytical chemistry, especially in techniques like LC-MS/MS []. They are added to samples, calibrators, and controls at a known concentration to correct for variations during sample preparation and instrument response. By comparing the signal of the target compound (Oleic Acid in this case) to the signal of the internal standard (this compound), researchers can achieve more accurate and reliable quantification of Oleic Acid in serum samples.
Disclaimer and Information on In-Vitro Research Products
Please be aware that all articles and product information presented on BenchChem are intended solely for informational purposes. The products available for purchase on BenchChem are specifically designed for in-vitro studies, which are conducted outside of living organisms. In-vitro studies, derived from the Latin term "in glass," involve experiments performed in controlled laboratory settings using cells or tissues. It is important to note that these products are not categorized as medicines or drugs, and they have not received approval from the FDA for the prevention, treatment, or cure of any medical condition, ailment, or disease. We must emphasize that any form of bodily introduction of these products into humans or animals is strictly prohibited by law. It is essential to adhere to these guidelines to ensure compliance with legal and ethical standards in research and experimentation.