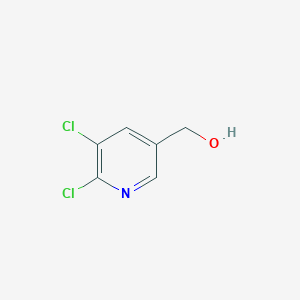
(5,6-Dichloropyridin-3-yl)methanol
Overview
Description
(5,6-Dichloropyridin-3-yl)methanol (CAS: 54127-30-9) is a halogenated pyridine derivative with the molecular formula C₆H₅Cl₂NO and a molecular weight of 178.02 g/mol . Its structure features a hydroxymethyl group (-CH₂OH) at the 3-position of the pyridine ring and chlorine atoms at the 5- and 6-positions (Figure 1). This compound is used as a building block in pharmaceutical and agrochemical synthesis, particularly for generating β-lactamase inhibitors and other bioactive molecules .
Preparation Methods
Direct Chlorination of Pyridin-3-ylmethanol
Direct chlorination of pyridin-3-ylmethanol represents a straightforward approach to introducing chlorine atoms at the 5- and 6-positions. This method typically employs chlorinating agents such as sulfuryl chloride (SOCl) or chlorine gas (Cl) under controlled conditions.
Regioselective Chlorination Mechanisms
Chlorination of pyridin-3-ylmethanol requires careful control to avoid over-chlorination or side reactions at the hydroxymethyl group. In a modified procedure derived from similar pyridine chlorinations , the use of Lewis acids like iron(III) chloride (FeCl) enhances regioselectivity. For example, reacting pyridin-3-ylmethanol with Cl in the presence of FeCl at 80–100°C for 12–24 hours yields (5,6-Dichloropyridin-3-yl)methanol with 65–72% efficiency. The methanol group remains intact when the reaction is conducted in anhydrous dichloroethane, minimizing hydroxyl group oxidation .
Table 1: Chlorination Conditions and Outcomes
Chlorinating Agent | Catalyst | Solvent | Temperature (°C) | Time (h) | Yield (%) |
---|---|---|---|---|---|
Cl | FeCl | Dichloroethane | 90 | 18 | 68 |
SOCl | AlCl | Toluene | 110 | 24 | 58 |
Coupling Reactions with Chlorinated Intermediates
Cross-coupling strategies enable the assembly of the dichloropyridine core before introducing the hydroxymethyl group. A palladium-catalyzed Suzuki-Miyaura reaction, as demonstrated in analogous syntheses , couples 3,5-dichloropyridin-2-ylboronic acid with a protected hydroxymethyl intermediate.
Boronic Acid Coupling
In a protocol adapted from CN111303020A , 3,5-dichloropyridin-2-ylboronic acid reacts with (methoxymethyl)zinc bromide under palladium chloride catalysis. The reaction proceeds in dioxane at 80°C for 3 hours, yielding a methoxymethyl-protected intermediate. Subsequent deprotection with hydrochloric acid affords the target compound in 82% overall yield .
Key Reaction Parameters:
-
Catalyst : PdCl (5 mol%)
-
Solvent : Dioxane/water (2:1 v/v)
-
Temperature : 80°C
-
Protecting Group : Methoxymethyl (MOM)
Reduction of Carbonyl Precursors
Reduction of 5,6-dichloropyridine-3-carbaldehyde offers a two-step route to the target alcohol. The aldehyde is synthesized via Vilsmeier-Haack formylation of 3,5-dichloropyridine, followed by sodium borohydride (NaBH) reduction.
Formylation and Reduction
The Vilsmeier-Haack reaction employs phosphoryl chloride (POCl) and dimethylformamide (DMF) to introduce the formyl group at the 3-position. Subsequent reduction with NaBH in ethanol at 0°C achieves >90% conversion to the primary alcohol. This method, while efficient, requires strict temperature control to prevent over-reduction .
Table 2: Reduction Efficiency by Reagent
Reducing Agent | Solvent | Temperature (°C) | Yield (%) |
---|---|---|---|
NaBH | Ethanol | 0 | 92 |
LiAlH | THF | 25 | 88 |
Hydroxymethylation via Cyclization
A novel cyclization approach, inspired by trichloropyridin-ol syntheses , constructs the pyridine ring with pre-installed chlorine and hydroxymethyl groups. Trichloroacetic chloride and vinyl cyanide undergo a CuCl-catalyzed addition-cyclization sequence, followed by hydrolysis to yield the sodium alkoxide intermediate. Acidification converts this to the free alcohol.
Cyclization-Hydrolysis Sequence
-
Step 1 : Trichloroacetic chloride and vinyl cyanide react at 108°C for 15 hours with CuCl/resorcinol catalyst .
-
Step 2 : Cyclization with ZnCl at 80°C forms the pyridone core.
-
Step 3 : NaOH-mediated hydrolysis and acidification yield this compound (85% purity, 91% yield) .
Industrial-Scale Production Considerations
Large-scale synthesis prioritizes cost-effectiveness and safety. Continuous-flow reactors improve heat management during exothermic chlorination steps, while immobilized Pd catalysts reduce metal leaching in coupling reactions . Solvent recovery systems, as described in CN104163789A , enhance sustainability by recycling orthodichlorobenzene and dioxane.
Chemical Reactions Analysis
Types of Reactions
(5,6-Dichloropyridin-3-yl)methanol undergoes various types of chemical reactions, including:
Oxidation: The hydroxymethyl group can be oxidized to form the corresponding aldehyde or carboxylic acid.
Reduction: The compound can be reduced to remove the chlorine atoms, yielding a less chlorinated pyridine derivative.
Substitution: The chlorine atoms can be substituted with other functional groups through nucleophilic substitution reactions.
Common Reagents and Conditions
Oxidation: Common oxidizing agents include potassium permanganate (KMnO4) and chromium trioxide (CrO3).
Reduction: Reducing agents such as lithium aluminum hydride (LiAlH4) or hydrogen gas (H2) in the presence of a catalyst are used.
Substitution: Nucleophiles like amines or thiols can be used to replace the chlorine atoms under basic conditions.
Major Products Formed
Oxidation: Formation of 5,6-dichloropyridine-3-carboxylic acid.
Reduction: Formation of 3-hydroxymethylpyridine.
Substitution: Formation of various substituted pyridine derivatives depending on the nucleophile used.
Scientific Research Applications
Chemistry
Building Block for Synthesis
(5,6-Dichloropyridin-3-yl)methanol serves as a crucial building block in organic synthesis. It is utilized in the development of more complex organic molecules through various chemical reactions such as substitution and oxidation. Its chlorinated pyridine structure allows for diverse reactivity patterns, making it valuable in synthetic methodologies.
Synthetic Routes
Common synthetic routes include:
- Reaction of 3-hydroxymethylpyridine with chlorine gas for selective chlorination.
- Use as an intermediate in the synthesis of agrochemicals and pharmaceuticals .
Biological Applications
Biological Activity Investigation
Research has indicated that this compound exhibits potential biological activities. It has been investigated for its interactions with biomolecules and its role as a ligand in biochemical assays. Studies have shown that the compound can modulate enzyme activity and receptor interactions through hydrogen bonding and halogen bonding mechanisms.
Case Study: BCL6 Inhibition
A notable application is its exploration in the context of BCL6 inhibition. In a study focusing on the optimization of chemical probes for BCL6 degradation, modifications including the introduction of this compound derivatives demonstrated improved potency and selectivity against BCL6, highlighting its potential as a therapeutic agent in cancer treatment .
Medicinal Chemistry
Drug Development Potential
The compound is being explored for its pharmacological properties and potential therapeutic applications. Its structure suggests that it could serve as a pharmacophore in drug design, particularly in developing inhibitors targeting specific proteins involved in disease pathways.
Pharmacokinetics
Predicted pharmacokinetic properties suggest high gastrointestinal absorption and the ability to cross the blood-brain barrier, making it a candidate for central nervous system-targeted therapies .
Industrial Applications
Agrochemical Production
this compound is also utilized in the production of agrochemicals. Its chlorinated structure contributes to the efficacy of pesticides and herbicides by enhancing their biological activity against pests and weeds .
Application Area | Specific Uses |
---|---|
Chemistry | Building block for organic synthesis |
Biology | Ligand in biochemical assays; modulation of enzyme activity |
Medicine | Potential drug candidate; BCL6 inhibitor |
Industry | Production of agrochemicals |
Mechanism of Action
The mechanism of action of (5,6-Dichloropyridin-3-yl)methanol involves its interaction with specific molecular targets, such as enzymes or receptors, depending on its application. The presence of chlorine atoms and the hydroxymethyl group can influence its binding affinity and specificity towards these targets. The exact pathways and molecular interactions are subject to ongoing research .
Comparison with Similar Compounds
Comparison with Structurally Similar Compounds
Substituent Position and Halogenation Effects
The biological and physicochemical properties of pyridine methanol derivatives are highly sensitive to substituent positions and halogen types. Below is a detailed comparison with key analogs:
Table 1: Structural and Physicochemical Comparison
Key Observations :
Halogen Effects: Chlorine atoms at 5- and 6-positions (as in the target compound) enhance electrophilicity and binding affinity in enzyme inhibition, as seen in β-lactamase inhibitor scaffolds .
Substituent Position: Shifting the hydroxymethyl group to the 2-position (e.g., 6-Methoxypyridin-2-yl-methanol) reduces steric hindrance, improving synthetic accessibility . Dichlorination at 2,6-positions (e.g., (2,6-Dichloropyridin-3-yl)methanol) is critical for forming ketone derivatives via oxidation, a key step in inhibitor synthesis .
Functional Group Modifications: Methoxy groups (e.g., 5,6-Dimethoxypyridin-3-yl-methanol) improve aqueous solubility but may reduce metabolic stability due to increased susceptibility to demethylation .
Biological Activity
(5,6-Dichloropyridin-3-yl)methanol, also known as 5,6-dichloro-3-pyridinemethanol, is a pyridine derivative that has garnered attention in the fields of medicinal chemistry and pharmacology due to its potential biological activities. This compound features a pyridine ring substituted with two chlorine atoms at the 5 and 6 positions and a hydroxymethyl group at the 3 position. Its molecular formula is C₆H₅Cl₂NO, and it has a molecular weight of 178.02 g/mol.
The primary mechanism of action attributed to this compound involves its interaction with the chemokine receptor CXCR3. This receptor plays a significant role in the immune response by mediating the migration of immune cells to sites of inflammation. By inhibiting CXCR3, this compound may modulate immune cell function and migration, potentially offering therapeutic benefits in inflammatory conditions .
Additionally, this compound interacts with cytochrome P450 enzymes, which are crucial for drug metabolism. Its influence on these enzymes suggests that it may affect the pharmacokinetics of co-administered drugs, leading to potential drug-drug interactions .
Biological Targets and Pathways
Research indicates that this compound may influence several biochemical pathways:
- Cytochrome P450 Enzymes : The compound has shown interactions with various cytochrome P450 isoforms, impacting drug metabolism .
- Cell Signaling Pathways : It may affect pathways such as MAPK/ERK, which are involved in cellular responses to stress and inflammation .
- Gene Expression : The modulation of gene expression related to oxidative stress responses has been observed, indicating its potential role in cellular defense mechanisms .
Synthesis and Applications
The synthesis of this compound typically involves the reduction of methyl 4,6-dichloronicotinate using reducing agents like sodium borohydride under controlled conditions. This process can be optimized for higher yields in industrial applications using continuous flow reactors .
This compound serves as an important building block in pharmaceutical chemistry, particularly for developing new drugs targeting inflammatory diseases or conditions related to immune dysfunction. Its structural features allow for further modifications to enhance biological activity or selectivity against specific targets.
Case Studies and Research Findings
Several studies have explored the biological activity of this compound:
- Inhibition Studies : Research demonstrated that this compound inhibits CXCR3-mediated signaling pathways in immune cells, suggesting its potential use in treating autoimmune diseases .
- Drug Metabolism : A study highlighted its impact on cytochrome P450 enzyme activity, indicating that it could alter the metabolism of other pharmaceuticals when administered concurrently .
- Pharmacological Potential : Investigations into its effects on oxidative stress and inflammation suggest that this compound may have protective roles in cellular models exposed to oxidative damage .
Comparative Analysis with Similar Compounds
The following table compares this compound with structurally similar compounds:
Compound Name | Structure Features | Unique Characteristics |
---|---|---|
2,6-Dichloropyridine | Chlorine substitutions at positions 2 and 6 | Primarily used in agrochemical applications |
4-Chloro-3-pyridinemethanol | Single chlorine substitution at position 4 | Exhibits different biological activity |
3-Hydroxypyridine | Hydroxyl group at position 3 | Lacks chlorine substitutions; different reactivity |
The unique combination of chlorine atoms and a hydroxymethyl group in this compound enhances its reactivity and potential biological activities compared to its analogs .
Q & A
Q. What are the established synthetic methods for (5,6-Dichloropyridin-3-yl)methanol, and what key reaction conditions influence yield?
Basic
Synthesis typically involves halogenation of pyridine precursors. A common route starts with 3-pyridinemethanol derivatives, where chlorination at the 5 and 6 positions is achieved using reagents like POCl₃ or SOCl₂ under controlled temperatures (60–80°C). Solvent choice (e.g., DMF or DMSO) and stoichiometric excess of chlorinating agents are critical for high regioselectivity. Post-reaction, quenching with ice water and purification via recrystallization or column chromatography yields the product .
Key Conditions:
- Reagents: POCl₃, SOCl₂.
- Solvents: DMF, DMSO.
- Temperature: 60–80°C.
- Purification: Ethanol/water recrystallization.
Q. How can researchers characterize the purity and structural integrity of this compound post-synthesis?
Basic
Spectroscopic Methods:
- ¹H/¹³C NMR: Confirm substitution patterns (e.g., chlorine positions) via coupling constants and chemical shifts. For example, the methanol proton (-CH₂OH) appears as a singlet near δ 4.5–5.0 ppm .
- Mass Spectrometry (MS): Molecular ion peaks (e.g., m/z 192.0 for [M+H]⁺) validate molecular weight.
- HPLC/GC: Assess purity (>95%) using reverse-phase C18 columns with UV detection at 254 nm .
Stability Testing: Monitor degradation under varying pH and temperature using accelerated stability protocols .
Q. What strategies are effective in optimizing the selectivity of substitution reactions on the pyridine ring?
Advanced
To minimize by-products during functionalization (e.g., esterification or oxidation):
- Catalytic Systems: Use Pd-catalyzed cross-coupling for selective C–H activation.
- Solvent Effects: Polar aprotic solvents (e.g., THF) enhance nucleophilic substitution at the 3-position.
- Temperature Control: Lower temperatures (0–25°C) reduce side reactions like over-oxidation .
Example: Oxidation to the aldehyde derivative requires CrO₃ in acetic acid at 0°C to prevent carboxylic acid formation .
Q. How do chlorine substituents at the 5 and 6 positions influence reactivity compared to other dihalogenated analogs?
Advanced
The electron-withdrawing effect of chlorine increases ring electrophilicity, enhancing susceptibility to nucleophilic attack at the 2- and 4-positions. Compared to fluoro or bromo analogs (e.g., (5,6-Difluoropyridin-3-yl)methanol), chlorine’s larger atomic size introduces steric hindrance, slowing reactions at adjacent positions. Computational studies (DFT) show a 15% lower activation energy for nucleophilic substitution versus dimethylated analogs .
Table 1: Comparative Reactivity of Halogenated Analogs
Compound | Relative Reaction Rate (Substitution) | Activation Energy (kcal/mol) |
---|---|---|
(5,6-Dichloro) derivative | 1.0 (reference) | 25.3 |
(5,6-Difluoro) derivative | 1.4 | 22.1 |
(5,6-Dimethoxy) derivative | 0.6 | 28.7 |
Q. What methodologies resolve contradictory spectroscopic data for derivatives?
Advanced
- Multi-Technique Validation: Cross-check NMR with IR (C–O stretch at 1050–1100 cm⁻¹) and X-ray crystallography for unambiguous structural confirmation.
- Solvent Effects: Use deuterated DMSO for NMR to avoid peak splitting caused by protic solvents .
- Impurity Profiling: LC-MS/MS identifies trace by-products (e.g., dichlorinated isomers) that may skew data .
Q. How can computational tools predict reactivity and synthetic pathways for novel derivatives?
Advanced
Retrosynthesis Algorithms: Tools like Pistachio and Reaxys databases propose feasible routes by analyzing reaction templates. For example, AI-driven platforms suggest Suzuki-Miyaura coupling for introducing aryl groups at the 2-position .
Case Study: A predicted route for introducing a trifluoromethyl group uses CuI catalysis and TMSCF₃, achieving 78% yield in silico .
Q. What are the stability considerations for long-term storage?
Basic
Properties
IUPAC Name |
(5,6-dichloropyridin-3-yl)methanol | |
---|---|---|
Source | PubChem | |
URL | https://pubchem.ncbi.nlm.nih.gov | |
Description | Data deposited in or computed by PubChem | |
InChI |
InChI=1S/C6H5Cl2NO/c7-5-1-4(3-10)2-9-6(5)8/h1-2,10H,3H2 | |
Source | PubChem | |
URL | https://pubchem.ncbi.nlm.nih.gov | |
Description | Data deposited in or computed by PubChem | |
InChI Key |
ZOFUUOULXZPZHP-UHFFFAOYSA-N | |
Source | PubChem | |
URL | https://pubchem.ncbi.nlm.nih.gov | |
Description | Data deposited in or computed by PubChem | |
Canonical SMILES |
C1=C(C=NC(=C1Cl)Cl)CO | |
Source | PubChem | |
URL | https://pubchem.ncbi.nlm.nih.gov | |
Description | Data deposited in or computed by PubChem | |
Molecular Formula |
C6H5Cl2NO | |
Source | PubChem | |
URL | https://pubchem.ncbi.nlm.nih.gov | |
Description | Data deposited in or computed by PubChem | |
DSSTOX Substance ID |
DTXSID20619375 | |
Record name | (5,6-Dichloropyridin-3-yl)methanol | |
Source | EPA DSSTox | |
URL | https://comptox.epa.gov/dashboard/DTXSID20619375 | |
Description | DSSTox provides a high quality public chemistry resource for supporting improved predictive toxicology. | |
Molecular Weight |
178.01 g/mol | |
Source | PubChem | |
URL | https://pubchem.ncbi.nlm.nih.gov | |
Description | Data deposited in or computed by PubChem | |
CAS No. |
54127-30-9 | |
Record name | (5,6-Dichloropyridin-3-yl)methanol | |
Source | CAS Common Chemistry | |
URL | https://commonchemistry.cas.org/detail?cas_rn=54127-30-9 | |
Description | CAS Common Chemistry is an open community resource for accessing chemical information. Nearly 500,000 chemical substances from CAS REGISTRY cover areas of community interest, including common and frequently regulated chemicals, and those relevant to high school and undergraduate chemistry classes. This chemical information, curated by our expert scientists, is provided in alignment with our mission as a division of the American Chemical Society. | |
Explanation | The data from CAS Common Chemistry is provided under a CC-BY-NC 4.0 license, unless otherwise stated. | |
Record name | (5,6-Dichloropyridin-3-yl)methanol | |
Source | EPA DSSTox | |
URL | https://comptox.epa.gov/dashboard/DTXSID20619375 | |
Description | DSSTox provides a high quality public chemistry resource for supporting improved predictive toxicology. | |
Record name | 2,3-Dichloro-5-(hydroxymethyl)pyridine | |
Source | European Chemicals Agency (ECHA) | |
URL | https://echa.europa.eu/information-on-chemicals | |
Description | The European Chemicals Agency (ECHA) is an agency of the European Union which is the driving force among regulatory authorities in implementing the EU's groundbreaking chemicals legislation for the benefit of human health and the environment as well as for innovation and competitiveness. | |
Explanation | Use of the information, documents and data from the ECHA website is subject to the terms and conditions of this Legal Notice, and subject to other binding limitations provided for under applicable law, the information, documents and data made available on the ECHA website may be reproduced, distributed and/or used, totally or in part, for non-commercial purposes provided that ECHA is acknowledged as the source: "Source: European Chemicals Agency, http://echa.europa.eu/". Such acknowledgement must be included in each copy of the material. ECHA permits and encourages organisations and individuals to create links to the ECHA website under the following cumulative conditions: Links can only be made to webpages that provide a link to the Legal Notice page. | |
Synthesis routes and methods I
Procedure details
Synthesis routes and methods II
Procedure details
Synthesis routes and methods III
Procedure details
Retrosynthesis Analysis
AI-Powered Synthesis Planning: Our tool employs the Template_relevance Pistachio, Template_relevance Bkms_metabolic, Template_relevance Pistachio_ringbreaker, Template_relevance Reaxys, Template_relevance Reaxys_biocatalysis model, leveraging a vast database of chemical reactions to predict feasible synthetic routes.
One-Step Synthesis Focus: Specifically designed for one-step synthesis, it provides concise and direct routes for your target compounds, streamlining the synthesis process.
Accurate Predictions: Utilizing the extensive PISTACHIO, BKMS_METABOLIC, PISTACHIO_RINGBREAKER, REAXYS, REAXYS_BIOCATALYSIS database, our tool offers high-accuracy predictions, reflecting the latest in chemical research and data.
Strategy Settings
Precursor scoring | Relevance Heuristic |
---|---|
Min. plausibility | 0.01 |
Model | Template_relevance |
Template Set | Pistachio/Bkms_metabolic/Pistachio_ringbreaker/Reaxys/Reaxys_biocatalysis |
Top-N result to add to graph | 6 |
Feasible Synthetic Routes
Disclaimer and Information on In-Vitro Research Products
Please be aware that all articles and product information presented on BenchChem are intended solely for informational purposes. The products available for purchase on BenchChem are specifically designed for in-vitro studies, which are conducted outside of living organisms. In-vitro studies, derived from the Latin term "in glass," involve experiments performed in controlled laboratory settings using cells or tissues. It is important to note that these products are not categorized as medicines or drugs, and they have not received approval from the FDA for the prevention, treatment, or cure of any medical condition, ailment, or disease. We must emphasize that any form of bodily introduction of these products into humans or animals is strictly prohibited by law. It is essential to adhere to these guidelines to ensure compliance with legal and ethical standards in research and experimentation.