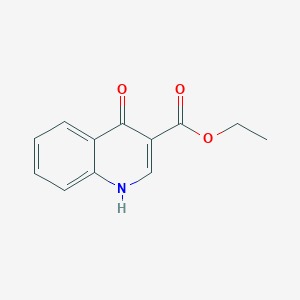
Ethyl 4-hydroxyquinoline-3-carboxylate
Overview
Description
Ethyl 4-hydroxyquinoline-3-carboxylate is a chemical compound with the molecular formula C12H11NO3. It is a derivative of quinoline, a heterocyclic aromatic organic compound.
Mechanism of Action
Target of Action
Quinoline derivatives have been known to interact with a variety of biological targets, including enzymes and receptors, which play crucial roles in various biochemical pathways .
Mode of Action
The Gould–Jacobs reaction is an organic synthesis for the preparation of quinolines and 4‐hydroxyquinoline derivatives . The mechanism for the Gould–Jacobs reaction begins with a nucleophilic attack from the amine nitrogen followed by the loss of ethanol to form the condensation product. A 6 electron cyclization reaction with the loss of another ethanol molecule forms a quinoline (ethyl 4-oxo-4,4a-dihydroquinoline-3-carboxylate) .
Biochemical Pathways
Quinoline derivatives are known to interact with various biochemical pathways, influencing cellular processes such as cell proliferation, apoptosis, and signal transduction .
Pharmacokinetics
The compound’s molecular weight (21722 Da) and structure suggest that it may have suitable properties for absorption and distribution within the body .
Result of Action
Quinoline derivatives have been reported to exhibit a range of biological activities, including antimicrobial, antimalarial, and anticancer effects .
Action Environment
The stability and efficacy of Ethyl 4-hydroxyquinoline-3-carboxylate can be influenced by various environmental factors. For instance, the compound should be stored at room temperature in an inert atmosphere for optimal stability . Additionally, factors such as pH, temperature, and the presence of other molecules can potentially affect the compound’s action .
Biochemical Analysis
Biochemical Properties
Quinoline derivatives are known to interact with various enzymes, proteins, and other biomolecules . The nature of these interactions can vary widely, depending on the specific structure of the quinoline derivative and the biomolecule it interacts with .
Cellular Effects
They can influence cell function by impacting cell signaling pathways, gene expression, and cellular metabolism .
Molecular Mechanism
The Gould–Jacobs reaction, a well-known method for the synthesis of quinoline derivatives, involves a nucleophilic attack from the amine nitrogen followed by the loss of ethanol to form a quinoline .
Metabolic Pathways
Quinoline and its derivatives are known to interact with various enzymes and cofactors, potentially affecting metabolic flux or metabolite levels .
Preparation Methods
Synthetic Routes and Reaction Conditions: Ethyl 4-hydroxyquinoline-3-carboxylate can be synthesized through several methods. One common method involves the reaction of aniline with diethyl ethoxymethylenemalonate, followed by cyclization to form the quinoline ring . The reaction typically requires a catalyst and specific reaction conditions, such as heating and the use of solvents like ethanol .
Industrial Production Methods: In industrial settings, the production of this compound often involves large-scale synthesis using similar methods but optimized for efficiency and yield. The use of continuous flow reactors and advanced purification techniques ensures the production of high-purity compounds .
Chemical Reactions Analysis
Types of Reactions: Ethyl 4-hydroxyquinoline-3-carboxylate undergoes various chemical reactions, including:
Oxidation: The compound can be oxidized to form quinoline derivatives with different functional groups.
Reduction: Reduction reactions can modify the quinoline ring, leading to the formation of different quinoline-based compounds.
Substitution: The hydroxyl group at the 4-position can be substituted with other functional groups using appropriate reagents.
Common Reagents and Conditions:
Oxidation: Common oxidizing agents include potassium permanganate and chromium trioxide.
Reduction: Reducing agents such as sodium borohydride and lithium aluminum hydride are often used.
Substitution: Reagents like alkyl halides and acyl chlorides are used for substitution reactions.
Major Products: The major products formed from these reactions depend on the specific reagents and conditions used. For example, oxidation can yield quinoline-4-carboxylic acid derivatives, while substitution can produce various quinoline esters .
Scientific Research Applications
Ethyl 4-hydroxyquinoline-3-carboxylate has a wide range of applications in scientific research:
Chemistry: It is used as a building block for the synthesis of more complex quinoline derivatives.
Biology: The compound has shown potential in biological studies, particularly in the development of antimicrobial and antiviral agents.
Medicine: Research has explored its use in developing drugs for various diseases, including cancer and infectious diseases.
Industry: It is used in the production of dyes, pigments, and other industrial chemicals
Comparison with Similar Compounds
- 4-Hydroxyquinoline-3-carboxylic acid
- Ethyl 7-bromo-4-hydroxyquinoline-3-carboxylate
- 8-Hydroxyquinoline
Comparison: Ethyl 4-hydroxyquinoline-3-carboxylate is unique due to its specific functional groups, which confer distinct chemical properties and reactivity. Compared to similar compounds, it may exhibit different biological activities and industrial applications. For instance, the presence of the ethyl ester group can influence its solubility and interaction with biological targets .
Biological Activity
Ethyl 4-hydroxyquinoline-3-carboxylate (E4HQ) is a compound of significant interest in medicinal chemistry due to its diverse biological activities. This article explores its synthesis, biological effects, mechanisms of action, and potential therapeutic applications, supported by recent research findings.
This compound has the molecular formula C₁₂H₁₁NO₃ and a molecular weight of approximately 217.22 g/mol. The compound is typically synthesized through methods such as the condensation of 4-hydroxyquinoline-3-carboxylic acid with ethanol in the presence of an acid catalyst or via cyclization reactions involving various precursors.
Biological Activities
Research indicates that E4HQ exhibits a range of biological activities, including:
The biological activity of E4HQ is attributed to its interaction with various biological targets:
- Enzyme Inhibition : Quinoline derivatives are known to inhibit enzymes involved in critical metabolic pathways, which may contribute to their antimicrobial and anticancer effects .
- Cell Signaling Modulation : E4HQ may influence cell signaling pathways that regulate apoptosis and cell proliferation. This modulation can lead to increased apoptosis in cancer cells, enhancing its anticancer potential .
- Reactive Oxygen Species (ROS) Generation : Some studies suggest that the cytotoxicity of quinoline derivatives may be linked to ROS overproduction, leading to oxidative stress in target cells .
Antimicrobial Study
A study evaluated the antimicrobial efficacy of E4HQ against common pathogens. The results indicated potent activity against Staphylococcus aureus and Escherichia coli, with inhibition zones comparable to standard antibiotics.
Bacterial Strain | Inhibition Zone (mm) | MIC (mg/mL) |
---|---|---|
Staphylococcus aureus | 24 | 0.5 |
Escherichia coli | 22 | 0.8 |
Klebsiella pneumoniae | 25 | 0.6 |
Anticancer Study
In vitro studies showed that E4HQ significantly inhibited the growth of various cancer cell lines:
Cell Line | IC₅₀ (mM) |
---|---|
MCF-7 | 14 |
A549 | 10 |
HL60 | 5 |
These findings suggest that E4HQ could serve as a lead compound for developing new anticancer therapies.
Properties
IUPAC Name |
ethyl 4-oxo-1H-quinoline-3-carboxylate | |
---|---|---|
Source | PubChem | |
URL | https://pubchem.ncbi.nlm.nih.gov | |
Description | Data deposited in or computed by PubChem | |
InChI |
InChI=1S/C12H11NO3/c1-2-16-12(15)9-7-13-10-6-4-3-5-8(10)11(9)14/h3-7H,2H2,1H3,(H,13,14) | |
Source | PubChem | |
URL | https://pubchem.ncbi.nlm.nih.gov | |
Description | Data deposited in or computed by PubChem | |
InChI Key |
YBEOYBKKSWUSBR-UHFFFAOYSA-N | |
Source | PubChem | |
URL | https://pubchem.ncbi.nlm.nih.gov | |
Description | Data deposited in or computed by PubChem | |
Canonical SMILES |
CCOC(=O)C1=CNC2=CC=CC=C2C1=O | |
Source | PubChem | |
URL | https://pubchem.ncbi.nlm.nih.gov | |
Description | Data deposited in or computed by PubChem | |
Molecular Formula |
C12H11NO3 | |
Source | PubChem | |
URL | https://pubchem.ncbi.nlm.nih.gov | |
Description | Data deposited in or computed by PubChem | |
DSSTOX Substance ID |
DTXSID10277822 | |
Record name | ethyl 4-hydroxyquinoline-3-carboxylate | |
Source | EPA DSSTox | |
URL | https://comptox.epa.gov/dashboard/DTXSID10277822 | |
Description | DSSTox provides a high quality public chemistry resource for supporting improved predictive toxicology. | |
Molecular Weight |
217.22 g/mol | |
Source | PubChem | |
URL | https://pubchem.ncbi.nlm.nih.gov | |
Description | Data deposited in or computed by PubChem | |
CAS No. |
52980-28-6, 26892-90-0 | |
Record name | Ethyl 4-oxo-1,4-dihydroquinoline-3-carboxylate | |
Source | CAS Common Chemistry | |
URL | https://commonchemistry.cas.org/detail?cas_rn=52980-28-6 | |
Description | CAS Common Chemistry is an open community resource for accessing chemical information. Nearly 500,000 chemical substances from CAS REGISTRY cover areas of community interest, including common and frequently regulated chemicals, and those relevant to high school and undergraduate chemistry classes. This chemical information, curated by our expert scientists, is provided in alignment with our mission as a division of the American Chemical Society. | |
Explanation | The data from CAS Common Chemistry is provided under a CC-BY-NC 4.0 license, unless otherwise stated. | |
Record name | Ethyl 4-oxo-1,4-dihydroquinoline-3-carboxylate | |
Source | ChemIDplus | |
URL | https://pubchem.ncbi.nlm.nih.gov/substance/?source=chemidplus&sourceid=0052980286 | |
Description | ChemIDplus is a free, web search system that provides access to the structure and nomenclature authority files used for the identification of chemical substances cited in National Library of Medicine (NLM) databases, including the TOXNET system. | |
Record name | 26892-90-0 | |
Source | DTP/NCI | |
URL | https://dtp.cancer.gov/dtpstandard/servlet/dwindex?searchtype=NSC&outputformat=html&searchlist=4345 | |
Description | The NCI Development Therapeutics Program (DTP) provides services and resources to the academic and private-sector research communities worldwide to facilitate the discovery and development of new cancer therapeutic agents. | |
Explanation | Unless otherwise indicated, all text within NCI products is free of copyright and may be reused without our permission. Credit the National Cancer Institute as the source. | |
Record name | ethyl 4-hydroxyquinoline-3-carboxylate | |
Source | EPA DSSTox | |
URL | https://comptox.epa.gov/dashboard/DTXSID10277822 | |
Description | DSSTox provides a high quality public chemistry resource for supporting improved predictive toxicology. | |
Record name | Ethyl 4-oxo-1,4-dihydroquinoline-3-carboxylate | |
Source | European Chemicals Agency (ECHA) | |
URL | https://echa.europa.eu/substance-information/-/substanceinfo/100.223.532 | |
Description | The European Chemicals Agency (ECHA) is an agency of the European Union which is the driving force among regulatory authorities in implementing the EU's groundbreaking chemicals legislation for the benefit of human health and the environment as well as for innovation and competitiveness. | |
Explanation | Use of the information, documents and data from the ECHA website is subject to the terms and conditions of this Legal Notice, and subject to other binding limitations provided for under applicable law, the information, documents and data made available on the ECHA website may be reproduced, distributed and/or used, totally or in part, for non-commercial purposes provided that ECHA is acknowledged as the source: "Source: European Chemicals Agency, http://echa.europa.eu/". Such acknowledgement must be included in each copy of the material. ECHA permits and encourages organisations and individuals to create links to the ECHA website under the following cumulative conditions: Links can only be made to webpages that provide a link to the Legal Notice page. | |
Record name | 4-HYDROXYQUINOLINE-3-CARBOXYLIC ACID ETHYL ESTER | |
Source | European Chemicals Agency (ECHA) | |
URL | https://echa.europa.eu/information-on-chemicals | |
Description | The European Chemicals Agency (ECHA) is an agency of the European Union which is the driving force among regulatory authorities in implementing the EU's groundbreaking chemicals legislation for the benefit of human health and the environment as well as for innovation and competitiveness. | |
Explanation | Use of the information, documents and data from the ECHA website is subject to the terms and conditions of this Legal Notice, and subject to other binding limitations provided for under applicable law, the information, documents and data made available on the ECHA website may be reproduced, distributed and/or used, totally or in part, for non-commercial purposes provided that ECHA is acknowledged as the source: "Source: European Chemicals Agency, http://echa.europa.eu/". Such acknowledgement must be included in each copy of the material. ECHA permits and encourages organisations and individuals to create links to the ECHA website under the following cumulative conditions: Links can only be made to webpages that provide a link to the Legal Notice page. | |
Record name | ETHYL 4-OXO-1,4-DIHYDROQUINOLINE-3-CARBOXYLATE | |
Source | FDA Global Substance Registration System (GSRS) | |
URL | https://gsrs.ncats.nih.gov/ginas/app/beta/substances/C69YF4B94W | |
Description | The FDA Global Substance Registration System (GSRS) enables the efficient and accurate exchange of information on what substances are in regulated products. Instead of relying on names, which vary across regulatory domains, countries, and regions, the GSRS knowledge base makes it possible for substances to be defined by standardized, scientific descriptions. | |
Explanation | Unless otherwise noted, the contents of the FDA website (www.fda.gov), both text and graphics, are not copyrighted. They are in the public domain and may be republished, reprinted and otherwise used freely by anyone without the need to obtain permission from FDA. Credit to the U.S. Food and Drug Administration as the source is appreciated but not required. | |
Synthesis routes and methods
Procedure details
Retrosynthesis Analysis
AI-Powered Synthesis Planning: Our tool employs the Template_relevance Pistachio, Template_relevance Bkms_metabolic, Template_relevance Pistachio_ringbreaker, Template_relevance Reaxys, Template_relevance Reaxys_biocatalysis model, leveraging a vast database of chemical reactions to predict feasible synthetic routes.
One-Step Synthesis Focus: Specifically designed for one-step synthesis, it provides concise and direct routes for your target compounds, streamlining the synthesis process.
Accurate Predictions: Utilizing the extensive PISTACHIO, BKMS_METABOLIC, PISTACHIO_RINGBREAKER, REAXYS, REAXYS_BIOCATALYSIS database, our tool offers high-accuracy predictions, reflecting the latest in chemical research and data.
Strategy Settings
Precursor scoring | Relevance Heuristic |
---|---|
Min. plausibility | 0.01 |
Model | Template_relevance |
Template Set | Pistachio/Bkms_metabolic/Pistachio_ringbreaker/Reaxys/Reaxys_biocatalysis |
Top-N result to add to graph | 6 |
Feasible Synthetic Routes
Q1: What is the significance of Ethyl 4-hydroxyquinoline-3-carboxylate in organic synthesis?
A1: this compound serves as a crucial intermediate in the multi-step synthesis of 4-hydroxyquinoline-3-carboxylic acid. [] This carboxylic acid derivative is a key intermediate in the production of Ivacaftor, a drug used to treat cystic fibrosis. []
Q2: How is this compound synthesized according to the provided research?
A2: The research outlines a three-step synthesis:
- Preparation of the precursor: Isatoic anhydrides are reacted with either ethyl malonate or ethyl acetoacetate to yield this compound derivatives. []
- Chlorination: The this compound derivative is then chlorinated using phosphorus oxychloride. []
- Condensation: The chlorinated product is condensed with hydrazine or its derivatives, resulting in the formation of new pyrazolo[4,3-c]quinolin-3-one derivatives. []
Disclaimer and Information on In-Vitro Research Products
Please be aware that all articles and product information presented on BenchChem are intended solely for informational purposes. The products available for purchase on BenchChem are specifically designed for in-vitro studies, which are conducted outside of living organisms. In-vitro studies, derived from the Latin term "in glass," involve experiments performed in controlled laboratory settings using cells or tissues. It is important to note that these products are not categorized as medicines or drugs, and they have not received approval from the FDA for the prevention, treatment, or cure of any medical condition, ailment, or disease. We must emphasize that any form of bodily introduction of these products into humans or animals is strictly prohibited by law. It is essential to adhere to these guidelines to ensure compliance with legal and ethical standards in research and experimentation.